Introduction to Wireless Power Transfer
Wireless Power Transfer (WPT) is a revolutionary technology that enables the transmission of electrical energy from a power source to a load without the need for physical connections or wires. This concept has gained significant attention in recent years due to its potential to simplify and enhance the functionality of various electronic devices and systems. In this article, we will explore the basic concepts behind wireless power transfer circuits, their applications, and the challenges associated with their implementation.
What is Wireless Power Transfer?
Wireless Power Transfer, also known as wireless energy transmission, is the process of transmitting electrical energy from a power source to a load without the use of wires or cables. This technology relies on the principles of electromagnetic induction, resonance, and electromagnetic radiation to transfer energy across a distance. WPT has the potential to revolutionize the way we power and charge electronic devices, making them more convenient, flexible, and efficient.
History of Wireless Power Transfer
The concept of wireless power transfer can be traced back to the late 19th century when Nikola Tesla, a renowned inventor and electrical engineer, demonstrated the possibility of transmitting electrical energy without wires. Tesla’s experiments involved the use of high-frequency alternating current (AC) to create a magnetic field that could induce an electric current in a receiver coil. Although Tesla’s work laid the foundation for WPT, it wasn’t until recent decades that significant advancements in technology have made practical applications of WPT feasible.
Principles of Wireless Power Transfer
Electromagnetic Induction
Electromagnetic induction is the fundamental principle behind most wireless power transfer systems. It involves the transfer of energy between two coils through a shared magnetic field. When an alternating current (AC) is passed through a primary coil, it generates a magnetic field that induces an electric current in a secondary coil placed in close proximity. This induced current can then be used to power a load or charge a battery.
Faraday’s Law of Induction
The electromagnetic induction phenomenon is governed by Faraday’s Law of Induction, which states that the electromotive force (EMF) induced in a conductor is proportional to the rate of change of the magnetic flux through the conductor. Mathematically, Faraday’s Law can be expressed as:
EMF = -N * (dΦ/dt)
Where:
– EMF is the electromotive force induced in the conductor (in volts)
– N is the number of turns in the coil
– Φ is the magnetic flux through the coil (in webers)
– t is time (in seconds)
The negative sign in the equation indicates that the induced EMF opposes the change in magnetic flux, as per Lenz’s Law.
Mutual Inductance
Mutual inductance is a key concept in wireless power transfer circuits. It represents the coupling between two coils and determines the efficiency of energy transfer. Mutual inductance (M) is defined as the ratio of the induced voltage in the secondary coil to the rate of change of current in the primary coil. It is expressed as:
M = (V_s) / (dI_p/dt)
Where:
– M is the mutual inductance (in henries)
– V_s is the induced voltage in the secondary coil (in volts)
– I_p is the current in the primary coil (in amperes)
– t is time (in seconds)
The higher the mutual inductance, the stronger the coupling between the coils and the more efficient the energy transfer.
Resonance
Resonance is another important principle in wireless power transfer. It occurs when the natural frequency of a system matches the frequency of an external stimulus, resulting in a significant increase in the amplitude of oscillations. In WPT systems, resonance is achieved by tuning the primary and secondary coils to the same resonant frequency, which allows for efficient energy transfer over longer distances.
Resonant Inductive Coupling
Resonant inductive coupling is a specific type of wireless power transfer that utilizes resonance to enhance the efficiency and range of energy transmission. In this method, both the primary and secondary coils are tuned to the same resonant frequency using capacitors. When the primary coil is energized with an AC current at the resonant frequency, it creates an oscillating magnetic field that induces a voltage in the secondary coil. Due to resonance, the energy transfer is highly efficient, even over larger distances compared to non-resonant inductive coupling.
Quality Factor (Q-factor)
The quality factor, or Q-factor, is a measure of the efficiency of a resonant system. It represents the ratio of the energy stored in the system to the energy dissipated per cycle. A higher Q-factor indicates a lower rate of energy dissipation and a more efficient resonant system. In wireless power transfer circuits, a high Q-factor is desirable to minimize losses and maximize the energy transfer efficiency.
The Q-factor of a resonant circuit can be calculated using the following formula:
Q = (2π * f * L) / R
Where:
– Q is the quality factor (dimensionless)
– f is the resonant frequency (in hertz)
– L is the inductance of the coil (in henries)
– R is the resistance of the coil (in ohms)
Electromagnetic Radiation
Electromagnetic radiation is another method of wireless power transfer that utilizes high-frequency electromagnetic waves to transmit energy. This approach is less common compared to inductive and resonant coupling due to its lower efficiency and potential health concerns associated with exposure to electromagnetic radiation.
Far-field Power Transfer
Far-field power transfer involves the use of electromagnetic waves in the far-field region, where the distance between the transmitter and receiver is much larger than the wavelength of the electromagnetic wave. In this region, the electromagnetic waves behave as plane waves and can propagate over long distances. However, the power density of the electromagnetic waves decreases with distance, making far-field power transfer less efficient compared to near-field methods like inductive and resonant coupling.
Rectenna
A rectenna, or rectifying antenna, is a device used in far-field wireless power transfer systems to convert the received electromagnetic waves into DC power. It consists of an antenna to capture the electromagnetic waves and a rectifier circuit to convert the high-frequency AC signal into DC power. Rectennas are commonly used in low-power applications, such as wireless sensor networks and radio-frequency identification (RFID) systems.
Wireless Power Transfer Circuit Design
Basic Circuit Components
A typical wireless power transfer circuit consists of the following basic components:
-
Power Source: The power source provides the electrical energy to be transmitted wirelessly. It can be a DC power supply, a battery, or an AC power source with appropriate rectification and filtering.
-
Oscillator: The oscillator generates the high-frequency AC signal that drives the primary coil. It determines the operating frequency of the WPT system and can be implemented using various circuit topologies, such as a Colpitts oscillator or a crystal oscillator.
-
Primary Coil: The primary coil is an inductor that generates the oscillating magnetic field when energized by the high-frequency AC signal from the oscillator. It is designed to have a specific inductance and resistance to achieve the desired resonant frequency and Q-factor.
-
Secondary Coil: The secondary coil is an inductor placed in close proximity to the primary coil. It captures the oscillating magnetic field generated by the primary coil and induces an AC voltage across its terminals. The secondary coil is also tuned to the same resonant frequency as the primary coil for efficient energy transfer.
-
Rectifier: The rectifier converts the induced AC voltage in the secondary coil into DC power. It typically consists of a bridge rectifier circuit made up of diodes or synchronous rectifiers (MOSFETs) for higher efficiency.
-
Voltage Regulator: The voltage regulator stabilizes the rectified DC voltage to provide a constant output voltage to the load. It can be a linear regulator or a switching regulator, depending on the power requirements and efficiency targets.
-
Load: The load is the electronic device or system that receives the wirelessly transmitted power. It can be a battery charging circuit, a motor, a sensor, or any other electrical load.
Resonant Circuit Design
Designing a resonant wireless power transfer circuit involves selecting the appropriate values for the inductors and capacitors to achieve the desired resonant frequency and Q-factor. The resonant frequency (f_r) of a series LC circuit is given by:
f_r = 1 / (2π * √(LC))
Where:
– f_r is the resonant frequency (in hertz)
– L is the inductance of the coil (in henries)
– C is the capacitance of the resonant capacitor (in farads)
To design a resonant circuit, follow these steps:
-
Choose the desired operating frequency (f_r) based on the application requirements and regulatory constraints.
-
Select the inductance (L) of the primary and secondary coils based on factors such as the desired power transfer distance, coil size, and coupling coefficient.
-
Calculate the required capacitance (C) using the resonant frequency equation:
C = 1 / (4π^2 * f_r^2 * L) -
Choose capacitors with the calculated capacitance value and appropriate voltage and current ratings.
-
Verify the resonant frequency and Q-factor of the designed circuit through simulations or experimental measurements.
Impedance Matching
Impedance matching is an important consideration in wireless power transfer circuits to maximize the power transfer efficiency and minimize reflections. The impedance of the primary and secondary coils should be matched to the impedance of the power source and load, respectively. This can be achieved through the use of impedance matching networks, such as LC networks or transformer-based networks.
The impedance matching network is designed to transform the impedance of the coil to match the impedance of the source or load. For example, a series capacitor can be added to the primary coil to cancel out its inductive reactance and match its impedance to the power source. Similarly, a parallel capacitor can be added to the secondary coil to match its impedance to the load.
Proper impedance matching ensures that the maximum power is delivered to the load and minimizes power losses due to reflections and impedance mismatches.
Coupling Factor
The coupling factor (k) is a measure of the magnetic coupling between the primary and secondary coils in a wireless power transfer system. It represents the fraction of the magnetic flux generated by the primary coil that is captured by the secondary coil. The coupling factor ranges from 0 to 1, with a higher value indicating stronger coupling and more efficient energy transfer.
The coupling factor can be calculated using the following formula:
k = M / √(L_p * L_s)
Where:
– k is the coupling factor (dimensionless)
– M is the mutual inductance between the coils (in henries)
– L_p is the inductance of the primary coil (in henries)
– L_s is the inductance of the secondary coil (in henries)
Factors that affect the coupling factor include:
-
Distance between the coils: As the distance between the primary and secondary coils increases, the coupling factor decreases.
-
Coil geometry: The size, shape, and number of turns of the coils influence the coupling factor. Larger coils with more turns generally have a higher coupling factor.
-
Alignment: Misalignment between the primary and secondary coils reduces the coupling factor. Perfect alignment results in maximum coupling.
-
Shielding and interference: Metallic objects and other electronic devices near the coils can interfere with the magnetic field and reduce the coupling factor.
Optimizing the coupling factor is crucial for achieving high efficiency in wireless power transfer systems. This can be done by carefully designing the coil geometry, minimizing the distance between the coils, ensuring proper alignment, and reducing interference from surrounding objects.
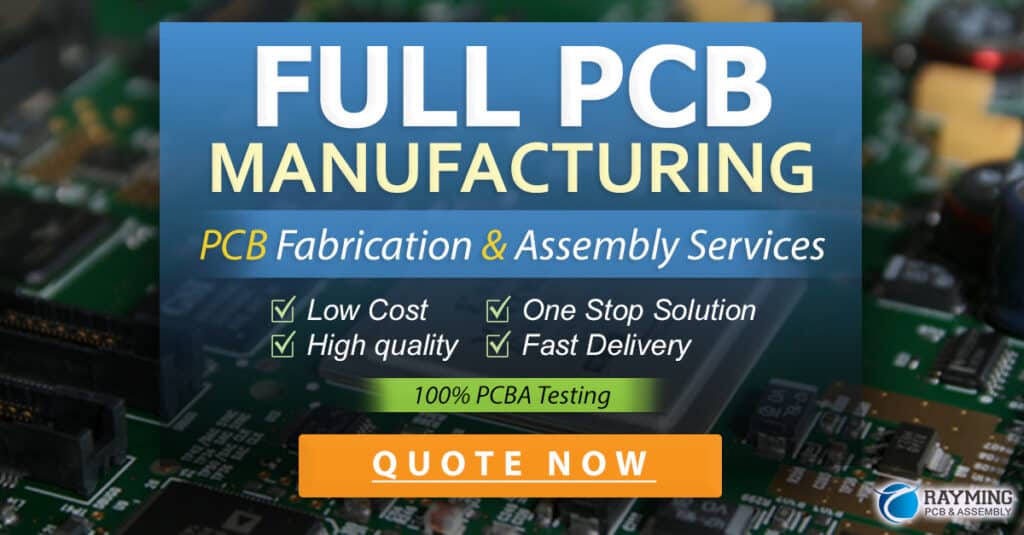
Applications of Wireless Power Transfer
Wireless power transfer has numerous applications across various industries and sectors. Some of the key applications include:
Consumer Electronics
WPT is increasingly being used in consumer electronic devices, such as smartphones, smartwatches, and wireless earbuds. Qi, a popular Wireless Charging standard, enables users to charge their devices by simply placing them on a wireless charging pad. This eliminates the need for cumbersome cables and makes charging more convenient.
Electric Vehicles
Wireless charging is being explored as a solution for charging electric vehicles (EVs). Instead of plugging in the vehicle to a charging station, WPT technology allows the vehicle to be charged wirelessly by parking it over a charging pad. This makes charging more convenient and eliminates the need for physical connectors, which can wear out over time.
Medical Devices
WPT has significant potential in the medical industry, particularly for implantable medical devices. Wireless charging can be used to power devices such as pacemakers, neurostimulators, and drug delivery systems without the need for surgical intervention to replace batteries. This improves patient comfort and reduces the risk of infections associated with battery replacement procedures.
Industrial Automation
WPT can be used in industrial automation to power sensors, actuators, and other devices in hard-to-reach or hazardous locations. This eliminates the need for wiring and enables greater flexibility in the placement of devices. WPT can also be used to charge mobile robots and drones, extending their operating time and reducing downtime for battery replacement.
Internet of Things (IoT)
The Internet of Things (IoT) relies on a vast network of connected sensors and devices. WPT can be used to power these devices wirelessly, eliminating the need for batteries or wired connections. This makes IoT devices more affordable, easier to deploy, and more environmentally friendly.
Challenges and Limitations
Despite the numerous benefits of wireless power transfer, there are several challenges and limitations that need to be addressed:
Efficiency
The efficiency of wireless power transfer systems is generally lower than that of wired systems due to power losses in the coils, rectifiers, and other circuit components. Improving efficiency is a key focus of research in WPT, with techniques such as resonant coupling, impedance matching, and synchronous rectification being explored to minimize losses.
Distance and Alignment
The efficiency of wireless power transfer decreases rapidly with increasing distance between the primary and secondary coils. This limits the practical range of WPT systems. Additionally, misalignment between the coils can significantly reduce the power transfer efficiency. Techniques such as adaptive tuning and multi-coil systems are being developed to improve the tolerance to misalignment and extend the effective range of WPT.
Safety and Health Concerns
Exposure to high-frequency electromagnetic fields generated by WPT systems has raised concerns about potential health risks. While the levels of electromagnetic radiation in most WPT systems are well below the safety limits set by regulatory agencies, long-term effects are still being studied. Shielding and other safety measures are being implemented to minimize exposure and ensure the safety of users.
Interference
WPT systems can potentially interfere with other electronic devices operating in the same frequency range. This can lead to performance degradation or malfunctioning of nearby devices. Proper shielding, frequency selection, and power control mechanisms are necessary to minimize interference and ensure compatibility with other electronic systems.
Standardization
The lack of globally accepted standards for wireless power transfer has hindered the widespread adoption of WPT technology. Different manufacturers have developed their own proprietary standards, leading to compatibility issues between devices. Efforts are being made to establish universal standards, such as the Qi standard for wireless charging, to ensure interoperability and promote the growth of the WPT market.
Future Developments
The field of wireless power transfer is rapidly evolving, with ongoing research and development aimed at addressing the challenges and expanding the applications of WPT technology. Some of the key areas of future development include:
Long-range WPT
Researchers are exploring techniques to extend the range of wireless power transfer beyond the current near-field limitations. Far-field power transfer using electromagnetic radiation, such as microwaves or lasers, is being investigated as a potential solution for long-range WPT. However, challenges related to efficiency, safety, and regulatory compliance need to be addressed.
Multi-device Charging
The ability to charge multiple devices simultaneously using a single WPT system is becoming increasingly important with the proliferation of portable electronic devices. Multi-coil systems and advanced control algorithms are being developed to enable efficient and flexible multi-device charging.
Dynamic Charging
Dynamic charging refers to the ability to charge devices while they are in motion. This is particularly relevant for electric vehicles, where wireless charging could be embedded in the road infrastructure, allowing vehicles to charge while driving. Dynamic charging could significantly extend the driving range of EVs and reduce the need for large onboard batteries.
Wireless Power Grids
The concept of wireless power grids envisions a future where WPT technology is integrated into the power distribution infrastructure. This would enable the wireless transmission of power over long distances, potentially revolutionizing the way electricity is generated, distributed, and consumed. However, significant technical and regulatory challenges need to be overcome to make wireless power grids a reality.
Conclusion
Wireless power transfer is a transformative technology that has the potential to revolutionize the way we power and charge electronic devices. By eliminating the need for physical connectors and cables, WPT offers convenience, flexibility, and improved user experience. The basic concepts behind wireless power transfer circuits, including electromagnetic induction, resonance, and electromagnetic radiation, provide the foundation for the development of efficient and practical WPT systems.
As research and development in WPT continue, we can expect to see significant advancements in efficiency, range, and functionality. The widespread adoption of wireless power transfer in consumer electronics, electric vehicles, medical devices, industrial automation, and the Internet of Things will drive innovation and shape the future of power delivery.
However, challenges related to efficiency, safety, interference, and standardization need to be addressed to fully realize the potential of wireless power transfer. Ongoing efforts in academia and industry are focused on developing solutions to these challenges and pushing the boundaries of WPT technology.
The future of wireless power transfer is exciting,
No responses yet