Introduction to Wideband RF
Wideband radio frequency (RF) technology has become increasingly important in modern electronic systems, enabling high-speed wireless communications, radar, and sensing applications. At the heart of these systems are the RF launches – the critical interface between the printed circuit board (PCB) and the antenna or other RF components. While RF launches may appear as simple footprints on a PCB, their design and optimization are crucial for achieving optimal system performance across a wide frequency range.
In this article, we will delve into the world of wideband RF launches, exploring their fundamental principles, design considerations, and best practices. We will discuss the challenges associated with high-frequency signal integrity, impedance matching, and loss minimization, and present practical techniques for optimizing RF launch performance. Moreover, we will highlight the importance of simulation and measurement in the design process and provide insights into emerging trends and future directions in wideband RF technology.
The Fundamentals of RF Launches
What is an RF Launch?
An RF launch is a specialized structure on a PCB that facilitates the transition of high-frequency signals between the board and an external component, such as an antenna, coaxial cable, or waveguide. The primary purpose of an RF launch is to efficiently couple the signal energy from the PCB trace to the external medium while minimizing reflections, losses, and electromagnetic interference (EMI).
RF launches come in various forms, depending on the specific application and frequency range. Some common types include:
- Coaxial connectors (SMA, SMB, SSMP, etc.)
- Waveguide transitions
- Microstrip-to-coplanar waveguide (CPW) transitions
- Stripline-to-coaxial transitions
The Importance of Impedance Matching
One of the key challenges in designing wideband RF launches is achieving proper impedance matching across the desired frequency range. Impedance mismatch occurs when there is a discontinuity in the characteristic impedance along the signal path, leading to reflections, standing waves, and reduced power transfer.
To minimize reflections and ensure maximum power transfer, the characteristic impedance of the RF launch must match that of the PCB trace and the external component. In most cases, a characteristic impedance of 50 ohms is used as a standard for RF systems.
Impedance matching is particularly challenging in wideband applications, as the frequency-dependent behavior of the materials and geometries can cause the impedance to vary significantly across the operating frequency range. Careful design and optimization of the RF launch structure are necessary to maintain a consistent impedance match over a wide bandwidth.
Signal Integrity Considerations
In addition to impedance matching, signal integrity is another critical aspect of RF launch design. As the operating frequency increases, the wavelength of the signal decreases, making the system more susceptible to losses, dispersion, and electromagnetic interference.
To maintain signal integrity, RF launches must be designed to minimize the following factors:
-
Conductor losses: At high frequencies, the skin effect causes the current to flow primarily on the surface of the conductors, leading to increased resistance and loss. Using low-loss materials, such as copper or silver, and optimizing the conductor geometry can help reduce conductor losses.
-
Dielectric losses: The dielectric substrate material used in the PCB can also contribute to signal loss, especially at higher frequencies. Low-loss dielectric materials, such as Rogers or Taconic laminates, are often used for RF applications to minimize dielectric losses.
-
Radiation losses: Discontinuities and asymmetries in the RF launch structure can cause unintended radiation, leading to signal loss and potential EMI issues. Proper shielding and grounding techniques, along with optimized launch geometries, can help minimize radiation losses.
-
Dispersion: Frequency-dependent propagation velocity can cause dispersion, leading to signal distortion and degradation. Using substrate materials with stable dielectric properties over the desired frequency range can help mitigate dispersion effects.
Design Considerations for Wideband RF Launches
Choosing the Right PCB Materials
The choice of PCB materials is crucial for the performance of wideband RF launches. The substrate material should have the following properties:
-
Low dielectric loss tangent: A low loss tangent ensures minimal dielectric losses, especially at higher frequencies.
-
Stable dielectric constant: A stable dielectric constant over the operating frequency range helps maintain consistent impedance and minimizes dispersion.
-
Controlled thickness: The substrate thickness affects the characteristic impedance and the cutoff frequency of the PCB traces. Thin substrates are generally preferred for higher frequency operation.
-
Low moisture absorption: Moisture absorption can alter the dielectric properties of the substrate, leading to performance degradation. Materials with low moisture absorption, such as PTFE-based laminates, are preferred for RF applications.
Some commonly used PCB materials for wideband RF launches include:
Material | Dielectric Constant | Loss Tangent | Typical Frequency Range |
---|---|---|---|
Rogers RO4003C | 3.38 | 0.0027 @ 10 GHz | DC to 40 GHz |
Rogers RO3003 | 3.00 | 0.0013 @ 10 GHz | DC to 77 GHz |
Taconic TLY-5 | 2.20 | 0.0009 @ 10 GHz | DC to 100 GHz |
Isola I-Tera | 3.45 | 0.0031 @ 10 GHz | DC to 40 GHz |
Optimizing the Launch Geometry
The geometry of the RF launch plays a significant role in its performance. The launch structure should be designed to provide a smooth transition between the PCB trace and the external component while maintaining a consistent characteristic impedance.
Some key geometrical considerations for RF launches include:
-
Trace width: The width of the PCB trace determines its characteristic impedance. Wider traces have lower impedance, while narrower traces have higher impedance. The trace width should be chosen to match the desired characteristic impedance, typically 50 ohms.
-
Trace thickness: The thickness of the PCB trace affects its current-carrying capacity and loss. Thicker traces have lower resistance and can handle higher currents but may also have higher capacitance. A balance between current-carrying capacity and loss should be considered when selecting the trace thickness.
-
Launch pad size: The size of the launch pad should be optimized to provide a good mechanical connection while minimizing parasitic capacitance and inductance. Larger pads may offer better mechanical stability but can also introduce unwanted discontinuities.
-
Ground plane clearance: The spacing between the launch pad and the surrounding ground plane affects the characteristic impedance and the coupling between the launch and the ground. Adequate ground plane clearance should be maintained to avoid impedance mismatch and excessive coupling.
-
Via design: Vias are often used to connect the launch pad to the inner layers of the PCB. The size, number, and placement of vias should be optimized to minimize inductance and ensure good electrical connection. Via-in-pad or buried via techniques can be used to improve the performance of the launch.
Simulation and Optimization
Given the complex nature of wideband RF launches, simulation is an essential tool for designing and optimizing their performance. Electromagnetic (EM) simulation software, such as Ansys HFSS, CST Studio Suite, or Keysight ADS, can be used to model the launch structure and predict its behavior across the desired frequency range.
EM simulation allows designers to:
- Verify the characteristic impedance of the launch and identify any mismatches.
- Evaluate the S-parameters of the launch, including return loss, insertion loss, and coupling.
- Visualize the electric and magnetic field distributions to identify potential issues, such as radiation or coupling.
- Optimize the launch geometry and material properties to achieve the desired performance.
Simulation-driven optimization techniques, such as parametric sweeps or genetic algorithms, can be used to find the optimal design parameters for the RF launch. These techniques involve running multiple simulations with varying parameters and evaluating the results against predefined performance metrics.
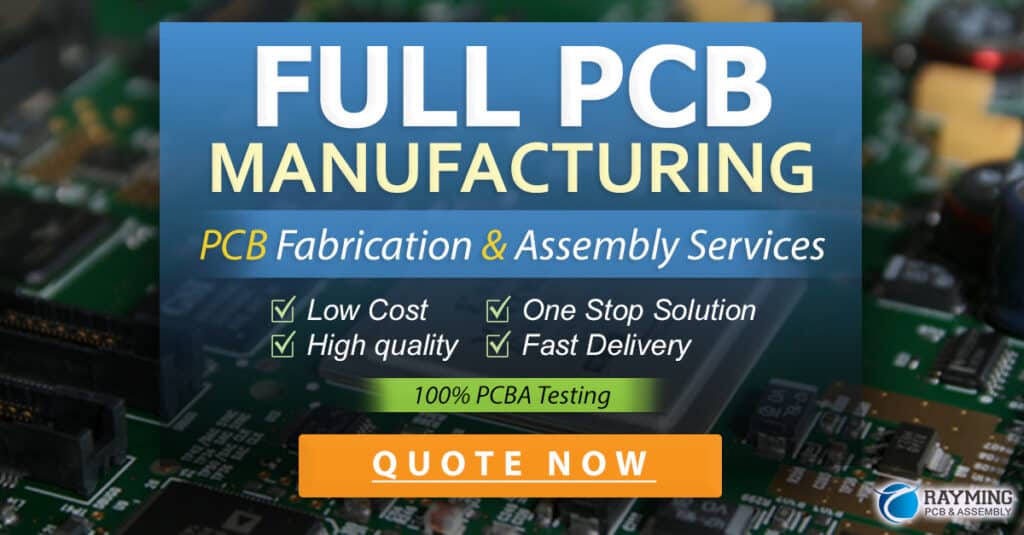
Measurement and Validation
While simulation is a powerful tool for designing wideband RF launches, measurement and validation are essential to ensure that the actual performance meets the desired specifications. Measuring the performance of RF launches requires specialized equipment, such as a vector network analyzer (VNA) and calibration standards.
The following steps are typically involved in measuring and validating RF launches:
-
Calibration: The VNA must be calibrated using appropriate standards, such as short, open, load, and through (SOLT) or through-reflect-line (TRL), to remove the effects of the measurement setup and cables.
-
Test fixture design: A test fixture is required to interface the RF launch with the VNA. The fixture should be designed to minimize any additional reflections or losses and provide a repeatable connection.
-
S-parameter measurement: The S-parameters of the RF launch are measured using the VNA across the desired frequency range. The measured S-parameters can be compared with the simulated results to validate the design.
-
Time-domain reflectometry (TDR): TDR measurements can be used to identify the location and nature of any discontinuities or impedance mismatches along the signal path. TDR can help diagnose issues such as poor via transitions or improper ground plane clearances.
-
Eye diagram analysis: For high-speed digital applications, eye diagram analysis can be used to evaluate the signal integrity of the RF launch. The eye diagram provides information about the signal’s rise time, fall time, jitter, and noise margin.
Measurement results should be carefully analyzed and compared with the simulation results and design specifications. Any discrepancies should be investigated and addressed through design iterations and optimization.
Best Practices for Wideband RF Launch Design
Based on the principles and considerations discussed above, here are some best practices for designing wideband RF launches:
- Choose low-loss, high-frequency PCB materials with stable dielectric properties.
- Optimize the trace width and thickness to achieve the desired characteristic impedance and minimize losses.
- Maintain adequate ground plane clearance around the launch pad to avoid impedance mismatch and coupling.
- Use via-in-pad or buried via techniques to minimize inductance and improve electrical connection.
- Implement proper shielding and grounding techniques to minimize radiation and EMI.
- Perform EM simulations to verify the launch performance and optimize the design.
- Validate the design through measurements and compare with simulation results.
- Follow IPC and industry standards for PCB design and manufacturing to ensure reliability and repeatability.
- Collaborate with PCB manufacturers and assembly houses to ensure the design is manufacturable and meets the required specifications.
- Document the design process, including simulation results, measurements, and any design trade-offs or decisions made.
Emerging Trends and Future Directions
As the demand for higher frequencies and bandwidths continues to grow, wideband RF launch technology is evolving to meet these challenges. Some emerging trends and future directions in this field include:
-
Millimeter-wave and terahertz frequencies: RF launches are being designed to operate at increasingly higher frequencies, such as millimeter-wave (30-300 GHz) and terahertz (0.1-10 THz) bands, enabling applications such as 5G, 6G, and high-resolution imaging.
-
Advanced materials and manufacturing techniques: New materials, such as low-temperature co-fired ceramics (LTCC), liquid crystal polymers (LCP), and 3D-printed dielectrics, are being explored for RF launch applications. These materials offer unique properties, such as low loss, high thermal conductivity, and the ability to create complex geometries.
-
Integrated and multi-functional launches: RF launches are being integrated with other components, such as filters, amplifiers, and antennas, to create multi-functional modules. This integration reduces the overall system size and improves performance by minimizing interconnect losses.
-
Advanced simulation and optimization techniques: Machine learning and artificial intelligence are being applied to EM simulation and optimization, enabling faster and more efficient design cycles. These techniques can help identify optimal design parameters and trade-offs based on a large dataset of simulations.
-
High-density interconnects (HDI): HDI technologies, such as microvias and embedded components, are being used to create compact and high-performance RF launches. HDI allows for shorter signal paths, reduced parasitics, and better control over impedance and coupling.
As wideband RF technology continues to advance, designers and engineers must stay up-to-date with the latest trends, techniques, and best practices to create high-performance, reliable, and cost-effective RF launches.
Frequently Asked Questions (FAQ)
-
What is the difference between an RF launch and a regular PCB footprint?
An RF launch is a specialized structure designed to facilitate the transition of high-frequency signals between a PCB and an external component, such as an antenna or coaxial cable. In contrast, a regular PCB footprint is a general-purpose pad or trace used for low-frequency or DC connections. RF launches require careful design and optimization to ensure proper impedance matching, low loss, and minimal signal distortion across a wide frequency range. -
Why is impedance matching important for RF launches?
Impedance matching is crucial for RF launches because it ensures maximum power transfer and minimizes reflections and standing waves. When there is an impedance mismatch between the PCB trace, the RF launch, and the external component, a portion of the signal power is reflected back, leading to reduced efficiency, signal distortion, and potential damage to the system. By matching the impedances, designers can optimize the performance of the RF launch and the overall system. -
What are some common challenges in designing wideband RF launches?
Some common challenges in designing wideband RF launches include: - Achieving consistent impedance matching across a wide frequency range
- Minimizing losses due to conductor, dielectric, and radiation effects
- Maintaining signal integrity and minimizing dispersion
- Selecting appropriate PCB materials with low loss and stable dielectric properties
- Optimizing the launch geometry for minimal parasitics and discontinuities
-
Ensuring manufacturability and reliability of the design
-
How can simulation help in the design of RF launches?
Electromagnetic (EM) simulation is a powerful tool for designing and optimizing RF launches. It allows designers to model the launch structure, predict its performance across the desired frequency range, and identify potential issues such as impedance mismatches, losses, or coupling. By using simulation, designers can iterate and optimize the design parameters, such as trace width, ground plane clearance, and via placement, to achieve the desired performance. Simulation also helps reduce the number of physical prototypes required, saving time and cost in the design process. -
What are some emerging trends in wideband RF launch technology?
Some emerging trends in wideband RF launch technology include: - Designing launches for millimeter-wave and terahertz frequencies
- Exploring advanced materials, such as LTCC, LCP, and 3D-printed dielectrics
- Integrating launches with other components to create multi-functional modules
- Applying machine learning and AI techniques for faster and more efficient simulation and optimization
- Using high-density interconnect (HDI) technologies for compact and high-performance launches
As wideband RF technology continues to evolve, staying informed about these trends and adopting best practices in design, simulation, and measurement will be essential for creating high-performance and reliable RF launches.
Conclusion
Wideband RF launches are critical components in modern electronic systems, enabling high-speed wireless communications, radar, and sensing applications. Designing high-performance RF launches requires a deep understanding of the principles of impedance matching, signal integrity, and loss minimization, as well as careful consideration of PCB materials, launch geometry, and manufacturing processes.
By following best practices in design, simulation, and measurement, engineers can create RF launches that meet the demanding requirements of wideband applications. As the technology continues to evolve, staying up-to-date with emerging trends and techniques will be essential for pushing the boundaries of RF performance and enabling new applications in 5G, 6G, and beyond.
No responses yet