Introduction to Thermal inertia
Thermal inertia is a property that describes a material’s ability to resist changes in temperature. It is a measure of how quickly a substance can absorb and release heat energy. Materials with high thermal inertia take longer to heat up and cool down, while those with low thermal inertia respond more rapidly to temperature changes.
The concept of thermal inertia is crucial in various fields, including engineering, architecture, geology, and planetary science. Understanding thermal inertia helps in designing energy-efficient buildings, studying Earth’s surface processes, and exploring other planets and moons in our solar system.
In this article, we will delve into the fundamentals of thermal inertia, its mathematical formulation, and its practical applications. We will also discuss the factors that influence thermal inertia and its effects on different systems.
What Is Thermal Inertia?
Thermal inertia is a measure of a material’s resistance to temperature change. It is determined by the material’s thermal conductivity, density, and specific heat capacity. Thermal inertia is expressed as the product of these three properties:
Thermal Inertia = (Thermal Conductivity × Density × Specific Heat Capacity)^(1/2)
- Thermal conductivity is the ability of a material to conduct heat. It is measured in watts per meter-kelvin (W/(m·K)).
- Density is the mass per unit volume of a material, typically expressed in kilograms per cubic meter (kg/m³).
- Specific heat capacity is the amount of heat required to raise the temperature of one kilogram of a substance by one degree Celsius. It is measured in joules per kilogram-kelvin (J/(kg·K)).
Materials with high thermal inertia have a combination of high thermal conductivity, high density, and high specific heat capacity. These materials can store and release large amounts of heat energy, making them resistant to temperature fluctuations. On the other hand, materials with low thermal inertia have low values for these properties and are more susceptible to temperature changes.
Factors Affecting Thermal Inertia
Several factors influence the thermal inertia of a material:
-
Composition: The chemical composition and structure of a material determine its thermal properties. For example, metals generally have higher thermal conductivity than non-metals, while porous materials have lower density and thermal conductivity compared to their solid counterparts.
-
Moisture content: The presence of water or other fluids in a material can significantly impact its thermal inertia. Water has a high specific heat capacity, meaning it can store a large amount of heat energy. As a result, moist materials tend to have higher thermal inertia than dry materials.
-
Temperature: The thermal properties of a material can vary with temperature. At higher temperatures, thermal conductivity and specific heat capacity may change, affecting the material’s thermal inertia.
-
Pressure: In some cases, pressure can influence a material’s thermal inertia by altering its density or thermal conductivity. For example, compressed gases have higher thermal conductivity than their uncompressed counterparts.
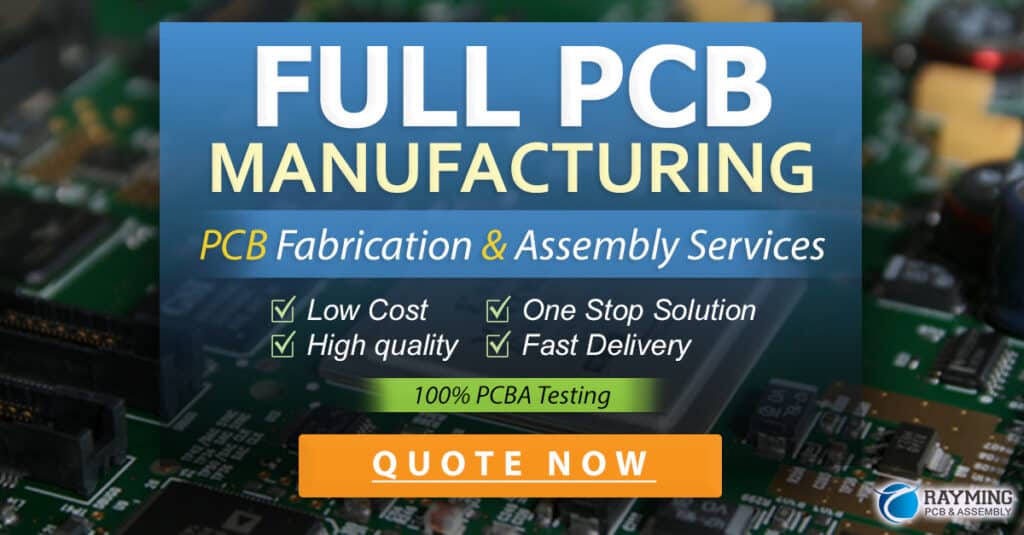
Applications of Thermal Inertia
Building and Construction
In the building and construction industry, thermal inertia plays a crucial role in designing energy-efficient structures. Materials with high thermal inertia, such as concrete and brick, are often used in construction to regulate indoor temperatures and reduce energy consumption for heating and cooling.
Thermal mass, which is closely related to thermal inertia, refers to a material’s ability to absorb, store, and release heat energy. Buildings with high thermal mass can maintain stable indoor temperatures by absorbing heat during the day and releasing it slowly at night, reducing the need for active heating and cooling systems.
Passive solar design techniques leverage thermal inertia to maximize the use of solar energy for heating and cooling. By strategically placing materials with high thermal inertia, such as concrete floors or walls, in areas exposed to direct sunlight, designers can create a natural heat storage system that regulates indoor temperatures.
Geology and Earth Sciences
Thermal inertia is an important concept in geology and Earth sciences, as it helps scientists understand the surface properties and processes of Earth and other planetary bodies.
Remote sensing techniques, such as satellite imaging, can measure the thermal inertia of Earth’s surface. By analyzing the temperature variations of different materials over a day-night cycle, researchers can infer the composition, moisture content, and physical properties of the surface.
For example, areas with high thermal inertia, such as bedrock or moist soils, exhibit smaller temperature variations between day and night compared to areas with low thermal inertia, such as dry sand or loose soil. This information is valuable for mapping surface geology, monitoring soil moisture, and studying land use patterns.
Thermal inertia also plays a role in understanding the formation and evolution of landscapes. Materials with high thermal inertia, such as rock or compacted soil, are more resistant to erosion and weathering processes compared to materials with low thermal inertia, such as loose sediments.
Planetary Science
In planetary science, thermal inertia is used to study the surface properties of planets, moons, and asteroids. By measuring the temperature variations of a planetary surface over time, scientists can infer its composition, physical properties, and potential for harboring life.
The thermal inertia of a planetary surface is influenced by factors such as grain size, rock abundance, and the presence of fluids. For example, the Moon has a low thermal inertia due to its fine-grained, dry regolith, while Mars has a higher thermal inertia in regions with exposed bedrock or ice.
Thermal inertia measurements have been crucial in identifying potential landing sites for spacecraft missions, as areas with high thermal inertia are more stable and less likely to have loose, dusty surfaces that could pose a risk to landing vehicles.
Effects of Thermal Inertia
Temperature Regulation
One of the primary effects of thermal inertia is its ability to regulate temperature fluctuations. Materials with high thermal inertia can absorb and store heat energy during periods of high temperature and release it slowly during periods of low temperature. This buffering effect helps to maintain more stable temperatures in a system.
In buildings, high thermal inertia materials can help to reduce indoor temperature fluctuations, making the space more comfortable for occupants and reducing the energy required for heating and cooling. In natural environments, high thermal inertia surfaces, such as rock or moist soil, experience smaller temperature variations compared to low thermal inertia surfaces, such as dry sand or snow.
Energy Efficiency
Thermal inertia can have a significant impact on energy efficiency, particularly in the built environment. By using materials with high thermal inertia in construction, designers can create buildings that are more energy-efficient and require less active heating and cooling.
Passive solar design strategies, which rely on thermal inertia, can reduce the energy consumption of buildings by leveraging the natural heat storage properties of materials. For example, a concrete floor exposed to direct sunlight can absorb heat during the day and release it slowly at night, reducing the need for mechanical heating.
In industrial processes, thermal inertia can be used to improve energy efficiency by reducing heat losses and minimizing temperature fluctuations. For example, in a furnace or boiler, using materials with high thermal inertia can help to maintain stable temperatures and reduce the energy required to maintain the desired operating conditions.
Climate and Weather
Thermal inertia plays a role in shaping Earth’s climate and weather patterns. The ocean, which has a high thermal inertia due to its large heat capacity, acts as a global temperature regulator. It absorbs heat during the summer months and releases it slowly during the winter, moderating seasonal temperature variations.
Land surfaces with high thermal inertia, such as moist soils or vegetated areas, also influence local climate and weather. These surfaces have a buffering effect on temperature fluctuations, reducing the intensity of heat waves and cold snaps.
In contrast, land surfaces with low thermal inertia, such as dry deserts or urban areas with extensive pavement, can amplify temperature extremes. These surfaces heat up rapidly during the day and cool down quickly at night, leading to larger diurnal temperature variations and potentially contributing to the urban heat island effect.
Geologic Processes
Thermal inertia influences various geologic processes, such as weathering, erosion, and landscape evolution. Materials with high thermal inertia, such as bedrock or consolidated sediments, are more resistant to temperature-driven weathering processes compared to materials with low thermal inertia, such as loose soil or unconsolidated sediments.
The differential weathering of materials based on their thermal inertia can shape the development of landforms over time. For example, in a mixed bedrock landscape, areas with high thermal inertia may form more prominent topographic features, such as ridges or cliffs, while areas with low thermal inertia may erode more readily, forming valleys or depressions.
Thermal inertia also affects the distribution of permafrost in cold regions. Areas with high thermal inertia, such as rock or ice-rich soil, are more likely to maintain stable permafrost conditions, while areas with low thermal inertia, such as dry, unconsolidated sediments, are more susceptible to permafrost thaw and degradation under changing climatic conditions.
Measuring and Mapping Thermal Inertia
Thermal inertia can be measured and mapped using various techniques, including remote sensing, in-situ measurements, and laboratory analysis.
Remote Sensing
Remote sensing is a powerful tool for measuring and mapping thermal inertia at different spatial scales. Satellites and airborne sensors can measure the surface temperature of Earth or other planetary bodies at multiple times during a day-night cycle. By analyzing the temperature variations over time, researchers can estimate the thermal inertia of the surface materials.
One common method for estimating thermal inertia from remote sensing data is the apparent thermal inertia (ATI) approach. ATI is calculated using the following formula:
ATI = (1 – A) / (ΔT × α)
Where:
– A is the albedo (reflectivity) of the surface
– ΔT is the diurnal temperature range (difference between maximum and minimum temperatures)
– α is a scaling factor that depends on the time of day and the surface properties
High ATI values indicate surfaces with high thermal inertia, while low ATI values indicate surfaces with low thermal inertia.
Thermal inertia mapping using remote sensing has been applied to various fields, including:
– Geology: Identifying surface composition, mapping bedrock and sediments, and studying landscape evolution
– Agriculture: Monitoring soil moisture, assessing crop health, and predicting irrigation requirements
– Urban planning: Identifying urban heat islands, assessing the thermal performance of buildings, and planning green infrastructure
In-situ Measurements
In-situ measurements involve directly measuring the thermal properties of materials using sensors or probes. These measurements provide detailed information about the thermal inertia of specific locations or samples.
Common in-situ measurement techniques include:
- Thermal conductivity probes: These devices measure the thermal conductivity of a material by applying a heat source and measuring the temperature response over time.
- Heat flux sensors: These sensors measure the rate of heat transfer through a material by measuring the temperature gradient and the thermal conductivity.
- Thermal imaging cameras: These cameras measure the surface temperature of a material and can be used to map the spatial distribution of thermal inertia.
In-situ measurements are often used to validate remote sensing data or to provide detailed information about the thermal properties of specific materials or sites.
Laboratory Analysis
Laboratory analysis involves measuring the thermal properties of materials under controlled conditions. Samples are collected from the field and analyzed using specialized equipment to determine their thermal conductivity, density, and specific heat capacity.
Common laboratory techniques for measuring thermal properties include:
- Guarded hot plate method: This method measures the thermal conductivity of a material by placing it between two temperature-controlled plates and measuring the heat flux through the sample.
- Differential scanning calorimetry (DSC): This technique measures the specific heat capacity of a material by comparing the heat flow to a sample and a reference material as they are heated or cooled.
- Pycnometry: This method measures the density of a material by determining its volume and mass.
Laboratory analysis provides accurate and precise measurements of thermal properties, which can be used to develop models or to validate field measurements.
Thermal Inertia in Urban Environments
Thermal inertia plays a significant role in urban environments, influencing the thermal comfort of city dwellers, energy consumption, and the urban heat island effect.
Urban Heat Island Effect
The urban heat island (UHI) effect refers to the phenomenon where urban areas experience higher temperatures compared to surrounding rural areas. This effect is caused by several factors, including the extensive use of heat-absorbing materials, such as concrete and asphalt, reduced vegetation cover, and anthropogenic heat sources, such as vehicles and air conditioning units.
Materials commonly found in urban environments, such as concrete, asphalt, and brick, have high thermal inertia. They absorb heat during the day and release it slowly at night, contributing to elevated nighttime temperatures. This can lead to increased energy consumption for cooling, reduced thermal comfort, and potential health risks during heat waves.
Mitigating the Urban Heat Island Effect
Several strategies can be employed to mitigate the urban heat island effect by leveraging the principles of thermal inertia:
-
Green infrastructure: Incorporating vegetation, such as trees, green roofs, and parks, into urban environments can help to reduce the UHI effect. Vegetation has a lower thermal inertia compared to built surfaces and can provide cooling through evapotranspiration and shading.
-
Cool roofs and pavements: Using materials with high solar reflectance and thermal emittance, such as white or light-colored roofs and pavements, can help to reduce heat absorption and lower surface temperatures. These materials have a lower thermal inertia and can help to mitigate the UHI effect.
-
Passive cooling strategies: Designing buildings with passive cooling features, such as natural ventilation, shading devices, and thermal mass, can help to reduce the reliance on mechanical cooling systems. By leveraging the thermal inertia of materials, these strategies can help to maintain comfortable indoor temperatures while reducing energy consumption.
-
Urban planning: Incorporating thermal inertia considerations into urban planning and zoning regulations can help to create more thermally comfortable and energy-efficient cities. This can include promoting the use of green infrastructure, encouraging the use of cool materials, and designing urban layouts that maximize shading and ventilation.
By understanding and addressing the role of thermal inertia in urban environments, city planners, architects, and policymakers can work towards creating more sustainable, resilient, and livable cities.
Frequently Asked Questions (FAQ)
-
What is thermal inertia, and why is it important?
Thermal inertia is a measure of a material’s ability to resist changes in temperature. It is determined by the material’s thermal conductivity, density, and specific heat capacity. Understanding thermal inertia is important for various applications, such as designing energy-efficient buildings, studying Earth’s surface processes, and exploring other planets and moons. -
What factors influence the thermal inertia of a material?
Several factors influence the thermal inertia of a material, including its composition, moisture content, temperature, and pressure. The chemical composition and structure of a material determine its thermal properties, while the presence of water or other fluids can significantly impact its thermal inertia. Temperature and pressure can also affect a material’s thermal conductivity and density, thereby influencing its thermal inertia. -
How does thermal inertia affect energy efficiency in buildings?
Thermal inertia can have a significant impact on energy efficiency in buildings. Materials with high thermal inertia, such as concrete and brick, can absorb and store heat energy during periods of high temperature and release it slowly during periods of low temperature. This buffering effect helps to maintain more stable indoor temperatures, reducing the energy required for heating and cooling. Passive solar design strategies, which rely on thermal inertia, can further enhance the energy efficiency of buildings. -
What role does thermal inertia play in shaping Earth’s climate and weather patterns?
Thermal inertia plays a crucial role in shaping Earth’s climate and weather patterns. The ocean, which has a high thermal inertia due to its large heat capacity, acts as a global temperature regulator, moderating seasonal temperature variations. Land surfaces with high thermal inertia, such as moist soils or vegetated areas, also influence local climate and weather by reducing the intensity of temperature fluctuations. In contrast, land surfaces with low thermal inertia can amplify temperature extremes and contribute to the urban heat island effect. -
How can the urban heat island effect be mitigated using thermal inertia principles?
The urban heat island effect can be mitigated by leveraging the principles of thermal inertia through various strategies. Incorporating green infrastructure, such as trees, green roofs, and parks, can help to reduce heat absorption and provide cooling through evapotranspiration and shading. Using cool roofs and pavements with high solar reflectance and thermal emittance can lower surface temperatures. Designing buildings with passive cooling features, such as natural ventilation, shading devices, and thermal mass, can maintain comfortable indoor temperatures while reducing energy consumption. Urban planning that considers thermal inertia can promote the use of green infrastructure, cool materials, and urban layouts that maximize shading and ventilation.
Conclusion
Thermal inertia is a fundamental property that describes a material’s ability to resist changes in temperature. It is determined by the material’s thermal conductivity, density, and specific heat capacity. Understanding thermal inertia is crucial for various applications, including building design, geology, and planetary science.
The effects of thermal inertia are far-reaching, influencing temperature regulation, energy efficiency, climate and weather patterns, and geologic processes. Materials with high thermal inertia, such as concrete and moist soils, can buffer temperature fluctuations and contribute to more stable thermal environments. In contrast, materials with low thermal inertia, such as dry sand and urban surfaces, can amplify temperature extremes and lead to increased energy consumption
No responses yet