Introduction to RF Amplifier Design
Radio Frequency (RF) amplifiers are essential components in modern wireless communication systems. They are used to increase the power of RF signals to a level suitable for transmission or further processing. Designing an efficient and reliable RF amplifier requires a deep understanding of the principles and techniques involved in the process.
In this comprehensive guide, we will delve into the intricacies of RF amplifier design, covering various aspects such as transistor selection, matching networks, stability considerations, and performance optimization. Whether you are a seasoned RF engineer or a curious enthusiast, this article will provide you with the knowledge and tools necessary to design high-performance RF amplifiers for your applications.
Key Considerations in RF Amplifier Design
Frequency Range and Bandwidth
The first step in designing an RF amplifier is to determine the frequency range and bandwidth of operation. This information will guide the selection of appropriate components and design techniques. RF amplifiers can be categorized into several types based on their frequency range:
- Low-Frequency (LF) Amplifiers: Operating from 30 kHz to 300 kHz
- Medium-Frequency (MF) Amplifiers: Operating from 300 kHz to 3 MHz
- High-Frequency (HF) Amplifiers: Operating from 3 MHz to 30 MHz
- Very High-Frequency (VHF) Amplifiers: Operating from 30 MHz to 300 MHz
- Ultra-High-Frequency (UHF) Amplifiers: Operating from 300 MHz to 3 GHz
- Microwave Amplifiers: Operating above 3 GHz
The bandwidth of an RF amplifier refers to the range of frequencies over which it can maintain a specified level of performance. Wideband amplifiers are designed to operate over a large frequency range, while narrowband amplifiers are optimized for a specific frequency or a narrow range of frequencies.
Power Output and Gain
The power output and gain requirements are crucial factors in RF amplifier design. The power output determines the maximum signal power that the amplifier can deliver to the load, while the gain represents the ratio of the output power to the input power.
RF amplifiers can be classified based on their power output levels:
- Low-Power Amplifiers: Typically less than 1 W
- Medium-Power Amplifiers: Ranging from 1 W to 10 W
- High-Power Amplifiers: Exceeding 10 W
The required gain of an RF amplifier depends on the application and the input signal level. It is essential to consider the trade-offs between gain, linearity, and efficiency when designing an RF amplifier.
Linearity and Efficiency
Linearity and efficiency are two critical aspects of RF amplifier performance. Linearity refers to the ability of an amplifier to produce an output signal that is a scaled version of the input signal without introducing distortion. Efficiency, on the other hand, measures the ratio of the output power to the DC power consumed by the amplifier.
There are several classes of RF amplifiers, each with different linearity and efficiency characteristics:
- Class A Amplifiers: High linearity but low efficiency (around 25-30%)
- Class B Amplifiers: Moderate linearity and efficiency (around 50-60%)
- Class AB Amplifiers: Compromise between Class A and Class B
- Class C Amplifiers: High efficiency but low linearity (efficiency can exceed 70%)
- Class D Amplifiers: High efficiency (up to 90%) but limited to low-frequency applications
- Class E and F Amplifiers: High efficiency (up to 90%) and suitable for high-frequency applications
Choosing the appropriate amplifier class depends on the specific requirements of the application, such as linearity, efficiency, and power output.
Transistor Selection for RF Amplifiers
Selecting the right transistor is a critical step in RF amplifier design. The transistor is the heart of the amplifier and plays a vital role in determining its performance characteristics. There are several factors to consider when choosing a transistor for an RF amplifier:
Frequency Range and Power Handling Capability
The transistor must be capable of operating at the desired frequency range and handling the required power levels. Different transistor technologies have different frequency and power handling capabilities:
- Silicon (Si) Transistors: Suitable for low to medium frequencies and power levels
- Gallium Arsenide (GaAs) Transistors: Suitable for high frequencies and medium to high power levels
- Gallium Nitride (GaN) Transistors: Suitable for high frequencies and high power levels
It is essential to refer to the transistor’s datasheet and specifications to ensure that it meets the requirements of the RF amplifier design.
Gain and Noise Figure
The gain and noise figure of the transistor are important parameters to consider. The gain determines the amplification provided by the transistor, while the noise figure indicates the amount of noise introduced by the transistor.
Low-noise transistors are preferred for RF amplifiers in receiver applications to minimize the noise added to the signal. High-gain transistors are desirable for transmitter applications to achieve the required output power levels.
Package and Thermal Considerations
The package of the transistor affects its thermal performance and ease of integration into the RF amplifier circuit. Common transistor packages for RF applications include:
- Surface-Mount Packages: SOT-23, SOT-89, QFN, etc.
- Leaded Packages: TO-92, TO-220, etc.
- Flange-Mount Packages: For high-power transistors
Proper heat dissipation is crucial for reliable operation of the RF amplifier. The thermal resistance of the transistor package and the design of the heat sink must be considered to ensure adequate thermal management.
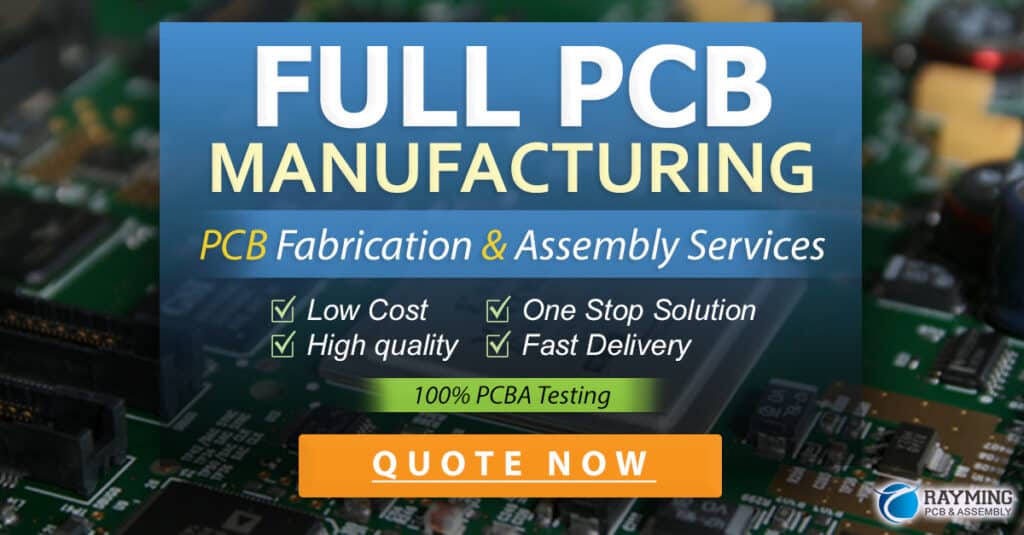
Matching Networks for RF Amplifiers
Matching networks play a vital role in RF amplifier design by ensuring maximum power transfer and minimizing reflections between the transistor and the input/output loads. Proper matching is essential for achieving optimal performance, efficiency, and stability of the RF amplifier.
Input and Output Matching
Input matching involves designing a network that matches the input impedance of the transistor to the source impedance (usually 50 ohms). This maximizes the power transfer from the source to the transistor and minimizes reflections.
Output matching involves designing a network that matches the output impedance of the transistor to the load impedance (usually 50 ohms). This maximizes the power transfer from the transistor to the load and minimizes reflections.
Matching Network Topologies
There are several matching network topologies commonly used in RF amplifier design:
- L-Network: Simple and compact, suitable for narrow-band matching
- Pi-Network: Provides additional flexibility and harmonic suppression
- T-Network: Offers improved bandwidth compared to L-network
- Transformer Matching: Useful for impedance transformation and DC isolation
The choice of matching network topology depends on the specific requirements of the RF amplifier, such as bandwidth, impedance transformation ratio, and harmonic suppression.
Simulation and Optimization
Matching networks are typically designed using simulation software such as Keysight ADS, NI AWR, or ANSYS HFSS. These tools allow for the optimization of the matching network components to achieve the desired performance metrics.
It is important to consider the effects of component tolerances, parasitics, and layout on the matching network performance. Sensitivity analysis and Monte Carlo simulations can be used to assess the robustness of the matching network design.
Stability Considerations in RF Amplifier Design
Stability is a critical aspect of RF amplifier design. An unstable amplifier can exhibit self-oscillation, which can lead to distortion, interference, and even damage to the circuit. Ensuring the stability of an RF amplifier is essential for reliable operation.
Stability Criteria
There are two main stability criteria used in RF amplifier design:
-
Unconditional Stability: An amplifier is unconditionally stable if it remains stable for all possible passive source and load impedances. This is the most desirable condition for an RF amplifier.
-
Conditional Stability: An amplifier is conditionally stable if it is stable for a specific range of source and load impedances. In this case, care must be taken to ensure that the amplifier operates within the stable region.
The stability of an RF amplifier can be assessed using various parameters such as the Rollett stability factor (K-factor), the Linvill stability factor (B1), and the Mu-factor.
Stability Improvement Techniques
If an RF amplifier is found to be unstable, several techniques can be employed to improve its stability:
- Resistive Loading: Adding resistive loading to the input or output of the amplifier can help dampen oscillations and improve stability.
- Neutralization: Neutralization involves introducing a feedback path to cancel out the internal feedback capacitance of the transistor, thereby improving stability.
- Feedback Compensation: Applying negative feedback can help reduce the gain and improve the stability of the amplifier.
- Bias Point Adjustment: Adjusting the bias point of the transistor can impact its stability characteristics. Proper biasing is crucial for stable operation.
It is important to perform stability analysis and implement appropriate stabilization techniques to ensure the reliable operation of the RF amplifier.
Performance Optimization and Testing
Once the RF amplifier design is complete, it is essential to optimize its performance and conduct thorough testing to validate its functionality and reliability.
Performance Metrics
Several key performance metrics are used to evaluate the performance of an RF amplifier:
- Gain: The ratio of the output power to the input power, usually expressed in dB.
- Linearity: The ability of the amplifier to produce an output signal that is a scaled version of the input signal without distortion. Metrics such as 1 dB compression point (P1dB) and third-order intercept point (IP3) are used to quantify linearity.
- Efficiency: The ratio of the output power to the DC power consumed by the amplifier. Power Added Efficiency (PAE) is a common metric used to evaluate the efficiency of RF amplifiers.
- Noise Figure: The amount of noise introduced by the amplifier, expressed in dB.
- Bandwidth: The range of frequencies over which the amplifier maintains a specified level of performance.
- Input and Output Return Loss: A measure of the matching quality at the input and output ports of the amplifier, expressed in dB.
Optimization Techniques
To optimize the performance of an RF amplifier, several techniques can be employed:
- Bias Point Optimization: Adjusting the bias point of the transistor can impact its gain, linearity, and efficiency. Finding the optimal bias point is crucial for achieving the desired performance.
- Harmonic Termination: Proper termination of harmonic frequencies can improve the efficiency and linearity of the amplifier. Harmonic termination networks can be designed to provide optimal impedances at harmonic frequencies.
- Linearization Techniques: Techniques such as predistortion, feedback, and feedforward can be used to improve the linearity of the amplifier, especially for high-power applications.
- Thermal Management: Proper thermal management is essential for reliable operation of the RF amplifier. Adequate heat sinking and cooling mechanisms should be employed to ensure that the transistor operates within its safe operating area.
Testing and Measurement
Thorough testing and measurement are crucial for validating the performance of the RF amplifier. Some common tests and measurements include:
- S-Parameter Measurement: Measuring the scattering parameters (S-parameters) of the amplifier using a vector network analyzer (VNA) to characterize its gain, matching, and stability.
- Power and Efficiency Measurement: Measuring the output power and efficiency of the amplifier using power meters and spectrum analyzers.
- Linearity Measurement: Measuring the 1 dB compression point (P1dB) and third-order intercept point (IP3) to assess the linearity of the amplifier.
- Noise Figure Measurement: Measuring the noise figure of the amplifier using a noise figure meter or a spectrum analyzer with a noise source.
- Spurious and Harmonic Measurement: Measuring the levels of spurious signals and harmonics generated by the amplifier using a spectrum analyzer.
Proper testing and measurement procedures should be followed to ensure accurate and reliable results. Calibration of the test equipment and proper handling of the RF amplifier during testing are essential for obtaining meaningful measurements.
Frequently Asked Questions (FAQ)
1. What is the difference between a low-noise amplifier (LNA) and a power amplifier (PA)?
A low-noise amplifier (LNA) is designed to amplify weak signals while introducing minimal noise. LNAs are typically used in receiver applications where the signal-to-noise ratio (SNR) is critical. On the other hand, a power amplifier (PA) is designed to provide high output power to drive antennas or other loads. PAs are used in transmitter applications where the focus is on delivering sufficient power to the load.
2. What is the purpose of matching networks in RF amplifier design?
Matching networks serve two main purposes in RF amplifier design:
1. Maximizing Power Transfer: Matching networks transform the impedances of the source and load to the optimal impedance of the transistor, ensuring maximum power transfer and minimizing reflections.
2. Improving Stability: Matching networks can be designed to provide the necessary impedances at the input and output of the transistor to ensure stable operation and prevent oscillations.
3. What are the advantages of using GaN transistors in RF amplifier design?
Gallium Nitride (GaN) transistors offer several advantages over traditional silicon (Si) and gallium arsenide (GaAs) transistors in RF amplifier design:
– High Breakdown Voltage: GaN transistors have a higher breakdown voltage, allowing for higher power handling capability and improved efficiency.
– Wide Bandgap: The wide bandgap of GaN enables higher operating temperatures and better thermal stability compared to Si and GaAs.
– High Electron Mobility: GaN transistors exhibit high electron mobility, resulting in faster switching speeds and higher frequency operation.
– High Power Density: GaN transistors can deliver higher power output in a smaller package size, making them suitable for compact and high-power RF amplifier designs.
4. What is the purpose of a heat sink in RF amplifier design?
A heat sink is a passive component used to dissipate heat generated by the transistor in an RF amplifier. It serves the following purposes:
– Thermal Management: The heat sink helps to transfer heat away from the transistor, preventing overheating and ensuring reliable operation.
– Temperature Regulation: By maintaining a stable operating temperature, the heat sink helps to minimize thermal variations that can affect the performance and stability of the amplifier.
– Improved Reliability: Proper heat sinking extends the lifespan of the transistor and other components by preventing thermal stress and degradation.
5. What are some common challenges in designing high-power RF amplifiers?
Designing high-power RF amplifiers presents several challenges, including:
– Thermal Management: Dissipating the large amount of heat generated by high-power transistors requires efficient thermal management techniques, such as advanced heat sinking and cooling mechanisms.
– Linearity vs. Efficiency Trade-off: Achieving high linearity and high efficiency simultaneously is challenging in high-power amplifiers. Techniques such as Doherty amplification and envelope tracking can be employed to improve the linearity-efficiency trade-off.
– Impedance Matching: Designing broadband impedance matching networks for high-power amplifiers can be complex due to the large impedance transformation ratios and the need to handle high currents and voltages.
– Device Parasitics: At high frequencies and power levels, device parasitics such as package inductance and capacitance can significantly impact the performance of the amplifier. Careful modeling and compensation techniques are required to mitigate their effects.
– Reliability and Robustness: Ensuring the reliability and robustness of high-power RF amplifiers is critical, especially in applications where long-term operation and harsh environmental conditions are encountered.
Conclusion
Designing high-performance RF amplifiers requires a comprehensive understanding of the principles, techniques, and challenges involved in the process. From selecting the appropriate transistor and designing matching networks to ensuring stability and optimizing performance, each step plays a crucial role in achieving the desired functionality and reliability.
This ultimate application guide has provided an in-depth exploration of the key aspects of RF amplifier design, empowering engineers and enthusiasts with the knowledge and tools necessary to tackle the complexities of this field.
As the demand for wireless communication systems continues to grow, the importance of RF amplifier design cannot be overstated. By mastering the art and science of RF amplifier design, engineers can contribute to the advancement of technologies that connect the world and shape the future of wireless communications.
[Table 1: Comparison of Transistor Technologies for RF Amplifiers]
| Transistor Technology | Frequency Range | Power Handling | Efficiency | Cost |
|———————-|—————–|—————-|————|——|
| Silicon (Si) | Low to Medium | Low to Medium | Moderate | Low |
| Gallium Arsenide (GaAs) | High | Medium to High | High | High |
| Gallium Nitride (GaN) | High | High | Very High | High |
[Table 2: Comparison of Amplifier Classes]
| Amplifier Class | Linearity | Efficiency | Applications |
|—————–|———–|————|————–|
| Class A | High | Low | Low-power, high-linearity |
| Class B | Moderate | Moderate | Medium-power, moderate linearity |
| Class AB | Moderate | Moderate | Compromise between Class A and B |
| Class C | Low | High | High-power, low linearity |
| Class D | High | Very High | Low-frequency, high-efficiency
No responses yet