What are 3D-printed electronics?
3D-printed electronics involve using various additive manufacturing methods to deposit conductive, insulating, and semiconducting materials in precise patterns to create electronic circuits and components. Some common techniques include:
-
Fused Deposition Modeling (FDM): Extruding molten thermoplastics through a nozzle to build up objects layer by layer. Conductive filaments can be used to print circuits.
-
Inkjet Printing: Depositing liquid inks containing conductive nanoparticles onto substrates to form circuits. Multiple print heads can deposit different materials.
-
Aerosol Jet Printing: Using a focused aerosol beam to deposit fine droplets of conductive inks onto substrates with high precision.
-
Stereolithography (SLA): Using UV light to selectively cure and harden photopolymer resins layer by layer. Conductive resins can be used to embed circuits.
The goal is to 3D print complete electronic devices with integrated circuits, sensors, antennas, batteries, and other components all in a single process. This could enable highly customized electronics for specific applications.
Promising Applications of 3D-Printed Electronics
Wearable and Implantable Devices
One of the most promising applications of 3D-printed electronics is in wearable and implantable medical devices. By 3D printing circuits onto flexible, biocompatible substrates, it’s possible to create sensors and stimulators that conform to the curves of the human body.
Some potential use cases include:
- Wearable health monitors that track vital signs
- Implantable neural probes and prosthetics
- Smart bandages and wound dressings with built-in sensors
- Transdermal drug delivery patches
The customizability of 3D printing could enable personalized devices that are tailored to an individual patient’s anatomy and medical needs. This could improve comfort, functionality, and patient outcomes.
Internet of Things (IoT) Devices
The Internet of Things (IoT) refers to the growing network of physical objects embedded with sensors, software, and connectivity, allowing them to collect and exchange data. 3D-printed electronics could enable the rapid prototyping and production of custom IoT devices for specific applications.
Some examples include:
- Industrial IoT sensors for monitoring equipment health and performance
- Agricultural IoT devices for precision farming
- Smart home devices like custom light fixtures and appliances
- Wearable IoT devices for fitness tracking and health monitoring
By 3D printing the enclosures and circuits for these devices in a single process, it’s possible to greatly speed up development cycles and create highly integrated, compact designs. The ability to produce devices on-demand could also reduce inventory costs and enable faster iteration.
Aerospace and Defense
The aerospace and defense industries have been early adopters of 3D printing technology for producing lightweight, complex parts. 3D-printed electronics could further extend these capabilities by enabling the creation of custom avionics, sensors, and communication devices.
Potential applications include:
- 3D-printed antennas and RF components
- Embedded sensors for structural health monitoring
- Bespoke control systems for drones and small satellites
- Ruggedized electronics for harsh environments
The ability to 3D print electronics could enable more integrated, compact designs that reduce weight and improve reliability. On-demand manufacturing could also simplify logistics and reduce lead times for critical components.
Rapid Prototyping and Product Development
Perhaps the most immediate application of 3D-printed electronics is in rapid prototyping and product development. By 3D printing functional prototypes with embedded circuits, designers can quickly test and iterate on new ideas.
Some benefits include:
- Faster design cycles with fewer assembly steps
- Ability to create complex geometries and consolidated parts
- Reduced tooling costs and lead times
- More opportunities for customization and experimentation
3D printing could democratize electronics development by lowering barriers to entry and enabling more individuals and small businesses to create custom devices. It could also accelerate innovation by allowing designers to take more risks and pursue novel ideas.
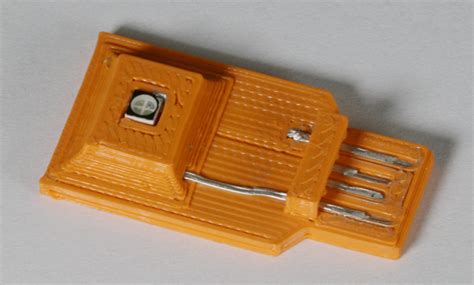
Challenges and Limitations
Despite the exciting potential of 3D-printed electronics, there are still significant challenges and limitations that must be addressed. Some of the key challenges include:
Material Properties and Performance
One of the biggest challenges is developing materials that have the necessary electrical and mechanical properties for high-performance electronics. Many current conductive inks and filaments have lower conductivity than traditional metals, which can limit performance.
Additionally, the layered structure of 3D-printed parts can create anisotropic properties, meaning the electrical and mechanical properties vary depending on the orientation of the layers. This can make it difficult to achieve consistent performance.
Researchers are working on developing new materials and printing techniques to improve conductivity, resolution, and isotropy. Some promising areas include:
- Nanoparticle inks with high conductivity
- Hybrid printing techniques that combine multiple materials
- Post-processing treatments to improve bonding and conductivity
- Conductive polymers and composites
However, more work is needed to develop materials that can match the performance of traditional electronics while also being compatible with 3D printing processes.
Resolution and Feature Size
Another challenge is achieving the high resolution and small feature sizes needed for many electronic applications. While some 3D printing techniques like aerosol jet printing can achieve resolutions down to 10 microns, this is still large compared to the nanoscale features in modern integrated circuits.
Additionally, the surface roughness and layer height of 3D-printed parts can limit the minimum feature size and create issues with signal integrity and electromagnetic interference.
Researchers are exploring ways to improve resolution and reduce surface roughness through techniques like:
- Hybrid printing with high-resolution inkjet and aerosol jet
- Two-photon polymerization for printing nanoscale features
- Smooth coatings and post-processing treatments
- Optimized print parameters and toolpath planning
However, achieving the resolution and feature sizes of traditional electronics manufacturing will likely require continued advances in materials, hardware, and software.
Integration and Packaging
Integrating 3D-printed electronics with other components and systems can also be challenging. Many applications require robust electrical connections, thermal management, and mechanical protection.
Printed circuits may need to interface with traditional PCBs, batteries, sensors, and other components. Ensuring reliable connections and avoiding signal integrity issues can be difficult with the irregular surfaces and geometries of 3D-printed parts.
Packaging and encapsulation are also important for protecting circuits from moisture, dust, and mechanical damage. Developing 3D printable packaging materials with the necessary barrier properties and compatibility with the printing process is an ongoing challenge.
Some potential solutions include:
- Co-design and co-optimization of printed and traditional components
- Embedded interconnects and vias for robust connections
- 3D-printed shielding and enclosures for EMI protection
- Conformal coatings and encapsulants for environmental protection
As 3D-printed electronics move from prototyping to production, developing standardized interfaces and packaging solutions will be critical for ensuring reliability and interoperability.
Scalability and Cost
Finally, there are challenges around scaling up 3D printing processes for volume production and ensuring cost-competitiveness with traditional manufacturing.
Many current 3D printing techniques are relatively slow and have limited throughput compared to mass production methods like injection molding and pick-and-place assembly. This can make them impractical or too expensive for high-volume applications.
Additionally, the cost of specialized 3D printing materials and equipment can be high compared to traditional materials and processes. This is especially true for applications that require exotic materials or very high resolution.
Some ways to improve scalability and cost include:
- Parallelization with multiple print heads and build platforms
- Continuous printing processes with automated material handling
- Hybrid processes that combine 3D printing with traditional manufacturing
- Standardization and economies of scale for materials and equipment
- Design optimization to reduce material usage and print time
As the technology matures and more companies adopt 3D-printed electronics, it’s likely that costs will come down and throughput will improve. However, it may take time for 3D printing to compete with traditional manufacturing for very high volume, low-cost applications.
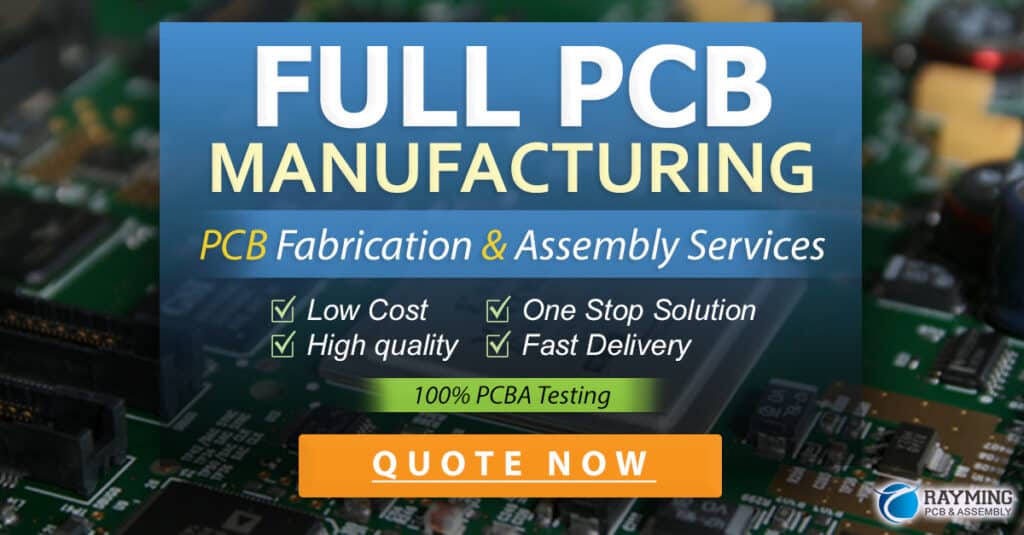
Frequently Asked Questions
What types of materials can be used for 3D-printed electronics?
A variety of materials can be used, including:
- Conductive thermoplastics (filaments)
- Metal nanoparticle inks
- Conductive polymers and composites
- Insulating and dielectric materials
- Semiconducting materials (e.g. silicon ink)
The choice of material depends on the specific application, printing process, and desired properties.
How does the performance of 3D-printed electronics compare to traditional electronics?
In general, 3D-printed electronics currently have lower performance than traditional electronics in terms of conductivity, speed, and power efficiency. However, they offer other benefits such as customizability, rapid prototyping, and the ability to create complex 3D structures.
As materials and printing processes improve, the performance gap is expected to narrow. Some 3D-printed devices have already demonstrated performance comparable to traditional electronics in certain applications.
What are some of the key companies and research institutions working on 3D-printed electronics?
Some notable companies and institutions include:
- Nano Dimension (3D-printed PCBs and antennas)
- Optomec (aerosol jet printing)
- Voxel8 (3D printing with conductive inks)
- PARC (printed sensors and flexible hybrid electronics)
- University of Minnesota (multi-material 3D printing)
- Singapore University of Technology and Design (SUTD) (3D-printed electronics research)
Many other companies, universities, and government labs are also actively researching and developing 3D-printed electronics technology.
What are the potential environmental benefits of 3D-printed electronics?
3D printing can potentially reduce waste and environmental impact compared to traditional electronics manufacturing by:
- Reducing material waste through additive manufacturing
- Enabling on-demand production to reduce overproduction and inventory
- Allowing for more compact and lightweight designs that use less material
- Facilitating the use of biodegradable and recyclable materials
- Reducing transportation emissions through distributed manufacturing
However, the environmental impact of 3D-printed electronics is still being studied, and there are concerns about the energy consumption of 3D printers and the recyclability of certain materials.
How long until 3D-printed electronics are widely used in consumer products?
It’s difficult to predict exactly when 3D-printed electronics will be widely used in consumer products, as it depends on many factors such as technological progress, market demand, and cost.
Some simple 3D-printed electronic devices like wearables and IoT sensors are already starting to enter the market, but more complex devices like smartphones and computers are likely further off.
Many experts believe that 3D-printed electronics will initially be used primarily for prototyping, low-volume production, and highly customized devices. As the technology matures and costs come down, we may start to see more widespread adoption in consumer products over the next 5-10 years.
However, it’s likely that 3D-printed electronics will coexist with traditional manufacturing methods for the foreseeable future, with each being used where it offers the most benefits in terms of cost, performance, and customization.
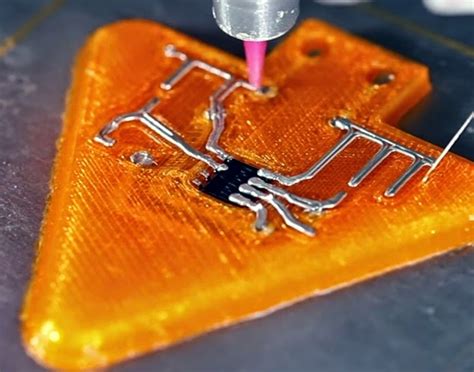
Conclusion
3D-printed electronics represent an exciting frontier in additive manufacturing, with the potential to revolutionize the way we design, prototype, and produce electronic devices. From personalized medical implants to custom IoT sensors, 3D printing could enable a new generation of innovative and highly customized electronic products.
However, significant challenges remain in terms of material properties, resolution, integration, and scalability. Researchers and companies are working hard to overcome these challenges through new materials, printing techniques, and design methodologies.
As the technology continues to advance, we can expect to see more and more 3D-printed electronic devices entering the market, initially in niche applications and then gradually expanding to more mainstream uses.
While it’s unlikely that 3D printing will completely replace traditional electronics manufacturing anytime soon, it offers a complementary approach that can enable new capabilities and accelerate innovation. As such, it’s an important technology to watch and one that could have a major impact on the future of electronics.
No responses yet