Introduction to Supercapacitors and Charging Circuits
Supercapacitors, also known as ultracapacitors or electric double-layer capacitors (EDLCs), are high-capacity energy storage devices that bridge the gap between traditional capacitors and rechargeable batteries. They offer a unique combination of high power density, rapid charging and discharging capabilities, and long cycle life, making them ideal for various applications, such as backup power systems, regenerative braking in electric vehicles, and energy harvesting.
To effectively utilize supercapacitors in these applications, it is essential to understand the fundamentals of Supercapacitor Charging circuits. This ultimate guide will provide an in-depth exploration of supercapacitor charging, covering the following topics:
- Supercapacitor basics and characteristics
- Charging methods and circuits
- Charging control and protection
- Practical considerations and applications
- Frequently asked questions (FAQ)
By the end of this guide, you will have a comprehensive understanding of supercapacitor charging circuits and be equipped with the knowledge to design and implement them in your projects.
Supercapacitor Basics and Characteristics
Before diving into the details of charging circuits, let’s first examine the fundamental principles and characteristics of supercapacitors.
How Supercapacitors Work
Supercapacitors store energy through the formation of an electric double layer at the interface between the electrode surface and the electrolyte. This double layer consists of two layers of charged ions, one positive and one negative, which are separated by a very thin insulating layer called the Helmholtz plane.
When a voltage is applied to the supercapacitor, ions in the electrolyte migrate towards the oppositely charged electrode, forming the electric double layer. The separation of charges in this layer creates a potential difference, allowing the supercapacitor to store energy.
Key Characteristics of Supercapacitors
Supercapacitors exhibit several unique characteristics that distinguish them from conventional capacitors and batteries:
-
High capacitance: Supercapacitors can achieve capacitance values ranging from a few farads to several thousand farads, which is orders of magnitude higher than conventional capacitors.
-
Low voltage: The rated voltage of a single supercapacitor cell is typically low, ranging from 2.3 V to 2.7 V. For higher voltage applications, multiple cells can be connected in series.
-
High power density: Supercapacitors can deliver and absorb high currents, enabling them to provide high power output in short bursts.
-
Fast charging and discharging: Due to their low internal resistance, supercapacitors can be charged and discharged very quickly, often in a matter of seconds.
-
Long cycle life: Supercapacitors can undergo hundreds of thousands of charge-discharge cycles without significant degradation in performance.
-
Wide operating temperature range: Supercapacitors can operate in a wide temperature range, typically from -40°C to +65°C, making them suitable for various environmental conditions.
Understanding these characteristics is crucial for designing effective supercapacitor charging circuits and selecting the appropriate components and control strategies.
Supercapacitor Charging Methods and Circuits
Charging supercapacitors requires careful consideration of their unique properties and the specific application requirements. In this section, we will explore different charging methods and their corresponding circuit implementations.
Constant Current (CC) Charging
Constant current (CC) charging is a simple and straightforward method for charging supercapacitors. In this method, a constant current is applied to the supercapacitor until it reaches its rated voltage. The charging time depends on the capacitance value and the charging current.
CC Charging Circuit
A basic CC charging circuit consists of a current-limiting resistor connected in series with the supercapacitor and a DC power source. The resistor value is chosen to limit the charging current to a safe level, typically specified in the supercapacitor’s datasheet.
The charging current (I) can be calculated using Ohm’s law:
I = (V_source – V_supercap) / R
Where:
– V_source is the DC power source voltage
– V_supercap is the supercapacitor’s initial voltage
– R is the current-limiting resistor value
The charging time (t) can be estimated using the following equation:
t = C × (V_rated – V_initial) / I
Where:
– C is the supercapacitor’s capacitance
– V_rated is the supercapacitor’s rated voltage
– V_initial is the supercapacitor’s initial voltage
– I is the charging current
Advantages and Disadvantages of CC Charging
Advantages:
– Simple and easy to implement
– Inexpensive, as it requires only a current-limiting resistor
– Suitable for low-power applications
Disadvantages:
– Slow charging, especially for large capacitance values
– Inefficient, as the current-limiting resistor dissipates power as heat
– No control over the charging voltage, which may lead to overcharging if not monitored
Constant Voltage (CV) Charging
Constant voltage (CV) charging involves applying a fixed voltage to the supercapacitor until it reaches its rated voltage. This method is more efficient than CC charging and provides better control over the charging voltage.
CV Charging Circuit
A basic CV charging circuit consists of a voltage regulator connected in series with the supercapacitor. The voltage regulator maintains a constant output voltage, ensuring that the supercapacitor is charged to its rated voltage without overcharging.
The charging current (I) can be calculated using the following equation:
I = (V_reg – V_supercap) / ESR
Where:
– V_reg is the voltage regulator’s output voltage
– V_supercap is the supercapacitor’s initial voltage
– ESR is the supercapacitor’s equivalent series resistance
The charging time (t) can be estimated using the same equation as in CC charging:
t = C × (V_rated – V_initial) / I
Advantages and Disadvantages of CV Charging
Advantages:
– More efficient than CC charging
– Provides control over the charging voltage, preventing overcharging
– Faster charging compared to CC charging
Disadvantages:
– Requires a voltage regulator, which increases circuit complexity and cost
– The charging current decreases exponentially as the supercapacitor approaches its rated voltage, resulting in longer charging times for the last 10-20% of the charge
CC-CV Charging
CC-CV charging combines the advantages of both CC and CV charging methods. In this method, the supercapacitor is initially charged with a constant current until it reaches a predetermined voltage threshold. Then, the charging switches to constant voltage mode, maintaining the voltage at the threshold level until the charging current drops below a specified value.
CC-CV Charging Circuit
A CC-CV charging circuit typically consists of a current-limiting resistor, a voltage regulator, and a switch or a diode to transition between CC and CV modes.
In the CC mode, the current-limiting resistor (R) determines the charging current. When the supercapacitor’s voltage reaches the voltage regulator’s output voltage (V_reg), the diode (D) becomes forward-biased, and the charging transitions to CV mode. The voltage regulator maintains the supercapacitor’s voltage at V_reg until the charging current drops below a specified threshold.
Advantages and Disadvantages of CC-CV Charging
Advantages:
– Combines the benefits of CC and CV charging
– Provides fast charging in the CC mode and precise voltage control in the CV mode
– Ensures that the supercapacitor is fully charged without overcharging
Disadvantages:
– More complex circuit design compared to CC or CV charging alone
– Requires additional components, such as a switch or a diode, increasing cost and size
Supercapacitor Charging Control and Protection
Proper control and protection are essential for safe and efficient supercapacitor charging. In this section, we will discuss various control and protection techniques that can be implemented in supercapacitor charging circuits.
Overcharge Protection
Overcharging a supercapacitor can lead to degradation of its performance and even cause permanent damage. To prevent overcharging, it is crucial to implement overcharge protection in the charging circuit.
Voltage Monitoring and Cut-Off
One common method for overcharge protection is to monitor the supercapacitor’s voltage and cut off the charging when it reaches a predetermined threshold. This can be achieved using a comparator or a microcontroller that continuously measures the supercapacitor’s voltage and triggers a switch or a relay to disconnect the charging source when the threshold is reached.
In this example, a comparator (U1) compares the supercapacitor’s voltage (V_supercap) with a reference voltage (V_ref) set by a voltage divider (R1 and R2). When V_supercap exceeds V_ref, the comparator’s output goes high, turning off the MOSFET (Q1) and disconnecting the charging source.
Balancing and Equalization
When multiple supercapacitor cells are connected in series to achieve higher voltage ratings, it is important to ensure that the cells are balanced and equalized during charging. Unbalanced cells can lead to overcharging of individual cells, reducing the overall lifespan of the supercapacitor bank.
Passive balancing techniques, such as using balancing resistors connected in parallel with each cell, can help equalize the voltage across the cells. Active balancing methods, such as using dedicated balancing ICs or circuits, provide more precise control over cell balancing and can be integrated into the charging circuit.
Overcurrent Protection
Overcurrent protection is necessary to prevent excessive current from flowing into the supercapacitor during charging, which can cause overheating and damage to the device.
Current Limiting
Current limiting can be achieved by using a current-limiting resistor, as discussed in the CC charging section. However, for more precise control and adjustability, a dedicated current-limiting circuit can be implemented.
One common approach is to use a current-sensing resistor in series with the supercapacitor and a comparator to monitor the voltage drop across the resistor. When the voltage drop exceeds a predetermined threshold, indicating an overcurrent condition, the comparator triggers a switch or a MOSFET to limit or shut off the charging current.
In this example, a current-sensing resistor (R_sense) is connected in series with the supercapacitor. The voltage drop across R_sense is monitored by a comparator (U1). When the voltage drop exceeds the reference voltage (V_ref), the comparator’s output goes high, turning off the MOSFET (Q1) and limiting the charging current.
Temperature Monitoring
Supercapacitors can generate heat during charging and discharging, especially at high currents. Excessive heat can degrade the performance and lifespan of the supercapacitor. Therefore, it is important to monitor the temperature of the supercapacitor and take appropriate actions to prevent overheating.
Temperature monitoring can be achieved by using temperature sensors, such as thermistors or integrated temperature sensors, placed in close proximity to the supercapacitor. The sensor’s output can be connected to a microcontroller or a dedicated temperature monitoring circuit that can trigger cooling mechanisms or shut off the charging when the temperature exceeds a safe limit.
In this example, a thermistor (R_th) is used to monitor the supercapacitor’s temperature. The thermistor’s resistance changes with temperature, causing a change in the voltage divider output (V_temp). A microcontroller (U1) measures V_temp and compares it with a predetermined temperature threshold. If the threshold is exceeded, the microcontroller can trigger a cooling fan or shut off the charging by controlling a MOSFET switch (Q1).
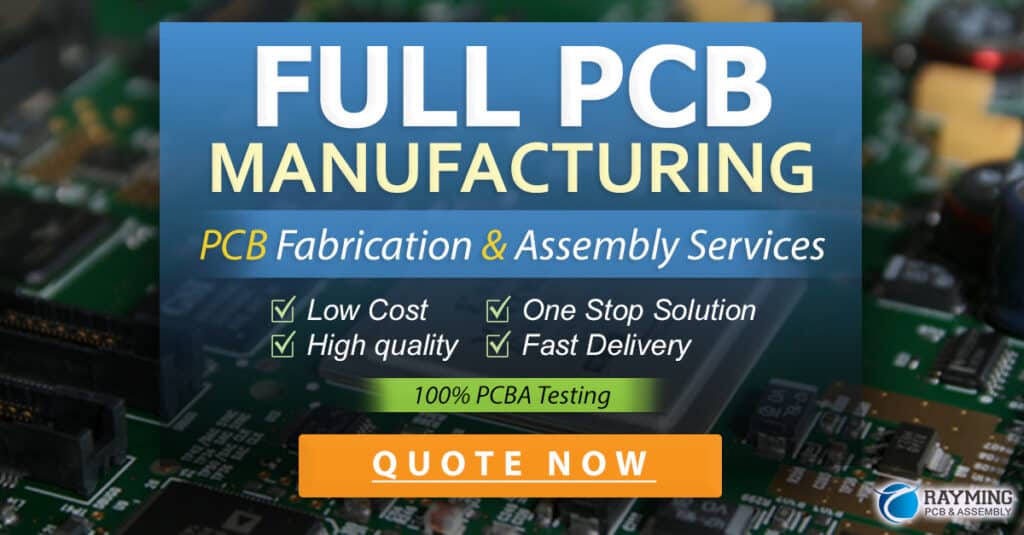
Practical Considerations and Applications
When designing and implementing supercapacitor charging circuits, several practical considerations should be taken into account to ensure optimal performance and reliability.
Selecting the Appropriate Supercapacitor
Choosing the right supercapacitor for a specific application involves considering factors such as capacitance, rated voltage, ESR, and physical size. The capacitance and rated voltage should be selected based on the energy storage requirements and the system voltage. The ESR should be as low as possible to minimize power losses during charging and discharging. The physical size of the supercapacitor should be compatible with the available space in the system.
Charging Source Considerations
The charging source should be capable of providing the required charging current and voltage for the supercapacitor. The source’s voltage should be regulated and stable to ensure consistent charging. In some cases, a dedicated charging IC or a power management unit may be necessary to provide the appropriate charging characteristics.
Parallel and Series Connections
For applications requiring higher capacitance or voltage ratings, supercapacitors can be connected in parallel or series.
When connecting supercapacitors in parallel, the total capacitance increases while the voltage rating remains the same. It is important to ensure that the supercapacitors have the same capacitance and voltage rating to prevent uneven current distribution and overcharging of individual cells.
When connecting supercapacitors in series, the voltage rating increases while the total capacitance decreases. Balancing and equalization techniques, as discussed earlier, should be implemented to prevent overcharging and ensure even voltage distribution across the cells.
Application Examples
Supercapacitor charging circuits find applications in various fields, such as:
-
Energy harvesting: Supercapacitors can be used to store energy from intermittent sources, such as solar panels or piezoelectric generators, and provide a stable power output to the load.
-
Backup power systems: Supercapacitors can provide short-term backup power during power outages or when the primary power source is disconnected.
-
Peak power buffering: In systems with high peak power demands, supercapacitors can be used to supplement the main power source and provide the necessary peak power, reducing the stress on the primary source.
-
Regenerative braking: In electric vehicles, supercapacitors can be charged during regenerative braking, storing the kinetic energy that would otherwise be lost as heat in conventional braking systems.
-
Pulse power applications: Supercapacitors can deliver high-power pulses in applications such as lasers, radar systems, and medical devices.
Frequently Asked Questions (FAQ)
-
What is the difference between a supercapacitor and a regular capacitor?
Supercapacitors have much higher capacitance values (typically in the range of farads) compared to regular capacitors (typically in the range of picofarads to microfarads). Supercapacitors also have lower voltage ratings and higher power density, allowing them to store and deliver large amounts of energy quickly. -
Can I charge a supercapacitor with a constant voltage source?
Yes, supercapacitors can be charged with a constant voltage source, such as a voltage regulator. However, it is important to ensure that the charging voltage does not exceed the supercapacitor’s rated voltage to prevent overcharging and damage. -
How long does it take to charge a supercapacitor?
The charging time of a supercapacitor depends on its capacitance, the charging current, and the initial and final voltages. In general, supercapacitors can be charged much faster than batteries, often in a matter of seconds or minutes. The charging time can be estimated using the equation: t = C × (V_rated – V_initial) / I, where C is the capacitance, V_rated is the rated voltage, V_initial is the initial voltage, and I is the charging current. -
What is the lifespan of a supercapacitor?
Supercapacitors have a much longer lifespan compared to batteries, often capable of undergoing hundreds of thousands of charge-discharge cycles without significant degradation in performance. The actual lifespan depends on factors such as the operating temperature, voltage, and current levels. Manufacturers typically provide estimated lifespans in the range of 10 to 20 years under nominal operating conditions. -
Can I use a supercapacitor to replace a battery in my application?
Supercapacitors can be used to replace batteries in certain applications, particularly those requiring high power density, fast charging, and long cycle life. However, supercapacitors have lower energy density compared to batteries, meaning they store less energy per unit volume. Therefore, the suitability of supercapacitors as a battery replacement depends on the specific energy storage and power requirements of the application. In some cases, a hybrid system combining supercapacitors and batteries may be the optimal solution.
Conclusion
Supercapacitor charging circuits play a crucial role in harnessing the unique properties of supercapacitors for various energy storage and power delivery applications. By understanding the fundamentals of supercapacitor charging, including charging methods, control techniques, and protection mechanisms, designers can create efficient and reliable charging systems that maximize the performance and lifespan of supercapacitors.
This ultimate guide has covered the key aspects of supercapacitor charging circuits, including:
- Supercapacitor basics and characteristics
- Charging methods, such as constant current (CC), constant voltage (CV), an
No responses yet