Introduction to Stripline and Microstrip Transmission Lines
In the world of high-frequency electronics, the choice of transmission line plays a crucial role in ensuring signal integrity, minimizing losses, and maintaining desired impedance. Two commonly used transmission line structures are stripline and microstrip. Both have their unique characteristics, advantages, and limitations, making them suitable for different applications. In this article, we will delve into the comparison between stripline and microstrip, examining their structures, properties, and performance in various aspects of high-frequency signal transmission.
What is a Stripline?
A stripline is a type of transmission line that consists of a flat conductor strip sandwiched between two parallel ground planes. The entire structure is embedded within a dielectric material, typically a printed circuit board (PCB) substrate. The stripline is designed to provide a controlled impedance environment for high-frequency signals, minimizing electromagnetic interference (EMI) and crosstalk.
What is a Microstrip?
A microstrip, on the other hand, is a transmission line structure that consists of a conductive strip placed on top of a dielectric substrate, with a ground plane on the opposite side of the substrate. Unlike stripline, the microstrip has one side exposed to air, making it more susceptible to external influences but also easier to access and modify.
Structural Differences between Stripline and Microstrip
Stripline Structure
The key structural features of a stripline include:
– Conductive strip sandwiched between two ground planes
– Dielectric material surrounding the conductive strip
– Symmetric cross-section
– Closed structure, providing shielding and isolation
Microstrip Structure
In contrast, the microstrip structure has the following characteristics:
– Conductive strip placed on top of a dielectric substrate
– Ground plane on the opposite side of the substrate
– Asymmetric cross-section
– Open structure, with one side exposed to air
Electromagnetic Field Distribution
Stripline Field Distribution
In a stripline, the electromagnetic field is confined within the dielectric material between the ground planes. The field lines are perpendicular to the conductive strip and the ground planes, resulting in a transverse electromagnetic (TEM) mode of propagation. This confinement of the field provides excellent isolation and reduces interference from external sources.
Microstrip Field Distribution
The electromagnetic field in a microstrip is not entirely confined within the dielectric substrate. A portion of the field lines extends into the air above the conductive strip. This results in a quasi-TEM mode of propagation, as the field is not purely transverse. The presence of the air-dielectric interface introduces some dispersion and losses, especially at higher frequencies.
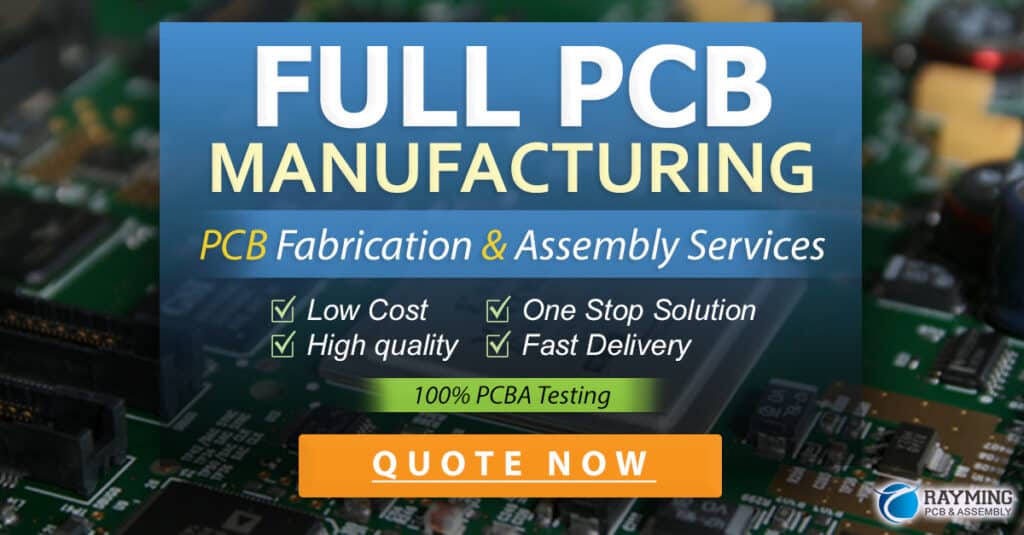
Characteristic Impedance
Stripline Characteristic Impedance
The characteristic impedance of a stripline depends on the width of the conductive strip, the thickness of the dielectric material, and the dielectric constant of the substrate. The symmetric structure of the stripline allows for a well-defined and controllable characteristic impedance. The impedance can be calculated using the following formula:
Z₀ = (60 / √ₑᵣ) × ln(4h / 0.67πw)
Where:
– Z₀ is the characteristic impedance
– ₑᵣ is the relative dielectric constant of the substrate
– h is the distance between the ground planes
– w is the width of the conductive strip
Microstrip Characteristic Impedance
The characteristic impedance of a microstrip is influenced by the width of the conductive strip, the thickness of the dielectric substrate, and the dielectric constant of the substrate. Due to the asymmetric structure and the presence of air, the calculation of the microstrip impedance is more complex and involves empirical formulas. One commonly used formula is:
Z₀ = (87 / √(ₑᵣ + 1.41)) × ln(5.98h / (0.8w + t))
Where:
– Z₀ is the characteristic impedance
– ₑᵣ is the relative dielectric constant of the substrate
– h is the thickness of the dielectric substrate
– w is the width of the conductive strip
– t is the thickness of the conductive strip
Dispersion and Losses
Stripline Dispersion and Losses
Stripline exhibits low dispersion and losses compared to microstrip. The symmetric structure and confinement of the electromagnetic field within the dielectric material contribute to its superior performance. The losses in stripline are primarily due to the dielectric loss tangent and the conductivity of the conductive strip and ground planes. Stripline is suitable for applications requiring low-loss transmission over a wide frequency range.
Microstrip Dispersion and Losses
Microstrip, due to its asymmetric structure and the presence of the air-dielectric interface, suffers from higher dispersion and losses compared to stripline. The quasi-TEM mode of propagation introduces dispersion, causing the signal to spread out in time as it travels along the microstrip. The losses in microstrip are attributed to dielectric losses, conductor losses, and radiation losses. The open structure of microstrip also makes it more susceptible to external interference and crosstalk.
Frequency Range and Bandwidth
Stripline Frequency Range and Bandwidth
Stripline is suitable for a wide frequency range, typically from a few megahertz to tens of gigahertz. The closed structure and low dispersion of stripline allow for a larger bandwidth compared to microstrip. The bandwidth of stripline is primarily limited by the dielectric losses and the frequency-dependent behavior of the substrate material.
Microstrip Frequency Range and Bandwidth
Microstrip is commonly used for frequencies up to several gigahertz. The open structure and higher dispersion of microstrip limit its bandwidth compared to stripline. The bandwidth of microstrip is also affected by the dielectric losses, conductor losses, and the presence of discontinuities or impedance mismatches along the transmission line.
EMI and Crosstalk
Stripline EMI and Crosstalk
Stripline provides excellent immunity to electromagnetic interference (EMI) and crosstalk due to its closed structure and confinement of the electromagnetic field within the dielectric material. The ground planes act as shields, preventing the penetration of external electromagnetic fields and minimizing the coupling between adjacent striplines. This makes stripline suitable for applications requiring high signal integrity and isolation.
Microstrip EMI and Crosstalk
Microstrip, with its open structure and exposed conductive strip, is more susceptible to EMI and crosstalk compared to stripline. The electromagnetic field extending into the air can interact with nearby microstrip lines or other components, leading to unwanted coupling and interference. Proper design techniques, such as maintaining adequate spacing between microstrip lines and using ground planes or shielding, can help mitigate EMI and crosstalk in microstrip circuits.
Manufacturing and Assembly
Stripline Manufacturing and Assembly
The manufacturing and assembly of stripline circuits require more complex processes compared to microstrip. The conductive strip needs to be embedded within the dielectric material, and the ground planes must be precisely aligned. Multilayer PCB fabrication techniques, such as lamination and plated through-holes, are commonly used for stripline manufacturing. The closed structure of stripline makes it more challenging to access and modify the circuit after fabrication.
Microstrip Manufacturing and Assembly
Microstrip circuits are relatively easier to manufacture and assemble compared to stripline. The conductive strip is placed on top of the dielectric substrate, and the ground plane is on the opposite side. Standard PCB fabrication techniques, such as etching and surface mount assembly, can be used for microstrip manufacturing. The open structure of microstrip allows for easy access and modification of the circuit, making it more flexible for prototyping and troubleshooting.
Applications
Stripline Applications
Stripline is widely used in applications that require low-loss, high-frequency signal transmission, such as:
– High-speed digital circuits
– Microwave filters and couplers
– Radar and communication systems
– Aerospace and defense electronics
– Test and measurement equipment
Microstrip Applications
Microstrip finds applications in various areas of high-frequency electronics, including:
– RF and microwave circuits
– Wireless communication systems
– Antenna design and integration
– Microwave sensors and detectors
– Consumer electronics and IoT devices
Comparison Table
Parameter | Stripline | Microstrip |
---|---|---|
Structure | Conductive strip sandwiched between two ground planes | Conductive strip on top of dielectric substrate, ground plane on the opposite side |
Field Distribution | TEM mode, confined within dielectric | Quasi-TEM mode, field extends into air |
Characteristic Impedance | Well-defined, controllable | Influenced by air-dielectric interface, more complex calculation |
Dispersion and Losses | Low dispersion and losses | Higher dispersion and losses |
Frequency Range and Bandwidth | Wide frequency range, larger bandwidth | Limited frequency range and bandwidth |
EMI and Crosstalk | Excellent immunity, minimal crosstalk | More susceptible to EMI and crosstalk |
Manufacturing and Assembly | Complex, multilayer PCB fabrication | Relatively easier, standard PCB fabrication |
Applications | High-speed digital circuits, microwave filters, radar systems | RF and microwave circuits, wireless communication, antenna design |
Frequently Asked Questions (FAQ)
1. When should I choose stripline over microstrip, or vice versa?
The choice between stripline and microstrip depends on the specific requirements of your application. If your design prioritizes low-loss transmission, wide bandwidth, and high signal integrity, stripline is often the preferred choice. On the other hand, if ease of manufacturing, access to the circuit, and flexibility are more important, microstrip may be a better fit.
2. Can I convert a stripline design to microstrip, or vice versa?
Converting between stripline and microstrip designs is possible, but it requires careful consideration of the differences in their characteristics and performance. The conversion process involves adjusting the dimensions of the conductive strip and dielectric substrate to achieve the desired impedance and signal integrity. It is recommended to perform simulations and tests to ensure the converted design meets the requirements.
3. How do I calculate the characteristic impedance of stripline and microstrip?
The characteristic impedance of stripline can be calculated using the formula:
Z₀ = (60 / √ₑᵣ) × ln(4h / 0.67πw)
For microstrip, the characteristic impedance can be approximated using the formula:
Z₀ = (87 / √(ₑᵣ + 1.41)) × ln(5.98h / (0.8w + t))
However, it is important to note that these formulas are simplified approximations, and more accurate calculations may require the use of electromagnetic simulation tools or specialized software.
4. What are the common dielectric materials used for stripline and microstrip?
Common dielectric materials used for stripline and microstrip include:
– FR-4: A glass-reinforced epoxy laminate, widely used for PCBs
– Rogers RO4000 series: High-frequency laminates with low dielectric loss and stable properties
– Teflon (PTFE): A low-loss dielectric material suitable for high-frequency applications
– Alumina (Al₂O₃): A ceramic substrate with excellent thermal and dielectric properties
The choice of dielectric material depends on factors such as the desired electrical properties, frequency range, thermal requirements, and cost.
5. How can I mitigate EMI and crosstalk in microstrip circuits?
To mitigate EMI and crosstalk in microstrip circuits, several techniques can be employed:
– Maintain adequate spacing between microstrip lines to reduce coupling
– Use ground planes or shielding to isolate sensitive signals
– Implement proper grounding and power supply decoupling techniques
– Use differential signaling to cancel out common-mode noise
– Apply filtering and impedance matching techniques to minimize reflections and interference
Careful layout and design practices, along with the use of electromagnetic simulation tools, can help optimize the performance of microstrip circuits in terms of EMI and crosstalk reduction.
Conclusion
In conclusion, stripline and microstrip are two fundamental transmission line structures used in high-frequency electronics. Understanding their differences in structure, electromagnetic field distribution, characteristic impedance, dispersion, losses, frequency range, bandwidth, EMI, crosstalk, manufacturing, and assembly is crucial for making informed decisions when designing high-frequency circuits.
Stripline offers superior performance in terms of low-loss transmission, wide bandwidth, and excellent immunity to EMI and crosstalk. Its closed structure and confinement of the electromagnetic field within the dielectric material make it suitable for applications demanding high signal integrity and isolation. However, stripline manufacturing and assembly are more complex, and accessing the circuit after fabrication can be challenging.
On the other hand, microstrip provides ease of manufacturing, accessibility, and flexibility. Its open structure and the presence of the air-dielectric interface make it more susceptible to dispersion, losses, EMI, and crosstalk compared to stripline. Microstrip is commonly used in RF and microwave circuits, wireless communication systems, and antenna design.
When choosing between stripline and microstrip, designers must carefully consider the specific requirements of their application, including signal integrity, bandwidth, loss tolerance, EMI sensitivity, manufacturing constraints, and cost. By understanding the strengths and limitations of each transmission line structure, designers can make informed decisions and optimize their high-frequency circuits for the desired performance and reliability.
As high-frequency electronics continue to advance, the demand for efficient and reliable signal transmission lines will only grow. Stripline and microstrip will remain essential tools in the designer’s toolkit, enabling the development of cutting-edge technologies across various industries, from telecommunications and aerospace to consumer electronics and beyond.
No responses yet