Introduction to Spin-Wave Memory
Spin-wave memory is an emerging technology that has the potential to revolutionize the field of data storage and processing. Unlike conventional electronic memory, which relies on the movement of electrons, spin-wave memory utilizes the collective oscillation of electron spins in magnetic materials. This unique approach offers several advantages, including ultra-low power consumption, non-volatility, and high-speed operation.
Recent breakthroughs in the field of spin-wave memory have brought this technology closer to practical implementation. Researchers have made significant progress in understanding the fundamental principles behind spin waves and developing novel materials and device architectures that can harness their potential.
What are Spin Waves?
Spin waves, also known as magnons, are collective excitations of electron spins in magnetic materials. In a ferromagnetic material, the spins of electrons tend to align in the same direction, creating a net magnetic moment. When an external stimulus, such as a magnetic field or an electrical current, is applied to the material, the spins can be excited and start to precess around their equilibrium position. This precession can propagate through the material in the form of a wave, which is called a spin wave.
Spin waves have several unique properties that make them attractive for memory applications:
- Low energy: Spin waves can be excited with very low energy inputs, making them suitable for ultra-low power devices.
- Non-volatility: The information stored in spin waves can be retained even when the power is turned off, enabling non-volatile memory.
- High speed: Spin waves can propagate at high speeds, potentially enabling fast read and write operations.
- Scalability: Spin waves can be confined to nanoscale dimensions, making them suitable for high-density memory devices.
Recent Breakthroughs in Spin-Wave Memory
Novel Materials for Spin-Wave Memory
One of the key challenges in developing spin-wave memory is finding suitable materials that can efficiently generate, propagate, and detect spin waves. Researchers have been exploring a wide range of magnetic materials, including ferromagnetic metals, ferrimagnetic insulators, and antiferromagnetic materials.
Material | Advantages | Challenges |
---|---|---|
Ferromagnetic metals | – Well-established fabrication processes – High spin-wave velocity |
– High damping – Limited spin-wave propagation distance |
Ferrimagnetic insulators | – Low damping – Long spin-wave propagation distance |
– Difficulty in generating and detecting spin waves |
Antiferromagnetic materials | – High spin-wave velocity – Robustness against external perturbations |
– Limited understanding of spin-wave dynamics – Difficulty in controlling spin waves |
Recent studies have shown promising results with ferrimagnetic insulators, such as yttrium iron garnet (YIG). YIG has extremely low damping, allowing spin waves to propagate over long distances without significant attenuation. Researchers have demonstrated the ability to generate, manipulate, and detect spin waves in YIG using a combination of microwave and optical techniques.
Advanced Device Architectures
In addition to exploring novel materials, researchers have been developing advanced device architectures that can efficiently harness the potential of spin waves for memory applications. One promising approach is the use of magnonic crystals, which are periodic structures that can control the propagation of spin waves.
Magnonic crystals can be designed to have band gaps, which are frequency ranges where spin waves cannot propagate. By tuning the geometry and material properties of the magnonic crystal, researchers can engineer the band structure to control the flow of spin waves and create memory devices.
Another approach is the use of spin-wave interferometers, which exploit the wave nature of spin waves to perform memory operations. In a spin-wave interferometer, two spin waves are generated and allowed to interfere with each other. The interference pattern can be used to encode and read out information, enabling memory functionality.
Integration with CMOS Technology
For spin-wave memory to become a practical reality, it needs to be compatible with existing complementary metal-oxide-semiconductor (CMOS) technology. CMOS is the dominant technology used in modern electronic devices, and any new memory technology must be able to integrate seamlessly with CMOS circuits.
Researchers have been working on developing hybrid spin-wave-CMOS devices that can leverage the advantages of both technologies. One approach is to use CMOS circuits to generate and detect spin waves, while using magnetic materials to store and process information. This hybrid approach can potentially offer the best of both worlds: the scalability and reliability of CMOS and the low-power, non-volatile operation of spin-wave memory.
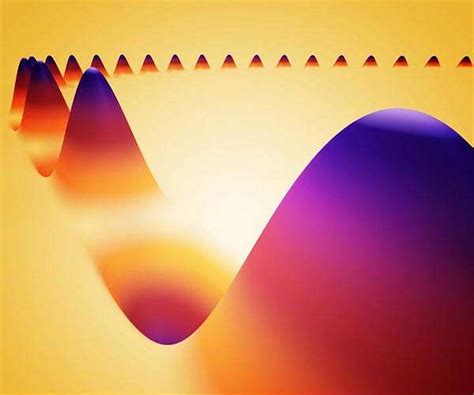
Challenges and Future Directions
Despite the significant progress made in spin-wave memory research, there are still several challenges that need to be addressed before this technology can be commercially viable.
Scalability
One of the main challenges is the scalability of spin-wave memory devices. While spin waves can be confined to nanoscale dimensions, the overall device size is often limited by the need for external components such as microwave generators and detectors. Researchers are working on developing more compact and integrated device architectures that can minimize the footprint of spin-wave memory.
Energy Efficiency
Although spin-wave memory has the potential for ultra-low power operation, the overall energy efficiency of the technology still needs to be improved. The energy required to generate and detect spin waves, as well as the energy dissipated during spin-wave propagation, must be minimized to make spin-wave memory competitive with other low-power memory technologies.
Speed and Bandwidth
The speed and bandwidth of spin-wave memory are currently limited by the time it takes for spin waves to propagate through the magnetic material. Researchers are exploring ways to increase the spin-wave velocity and reduce the propagation time, such as using materials with higher spin-wave velocities or designing device architectures that minimize the propagation distance.
Reliability and Endurance
The reliability and endurance of spin-wave memory devices are critical factors for practical applications. The magnetic materials and device structures must be able to withstand repeated read and write operations without degradation or failure. Researchers are investigating the long-term stability and reliability of spin-wave memory devices and developing strategies to improve their endurance.
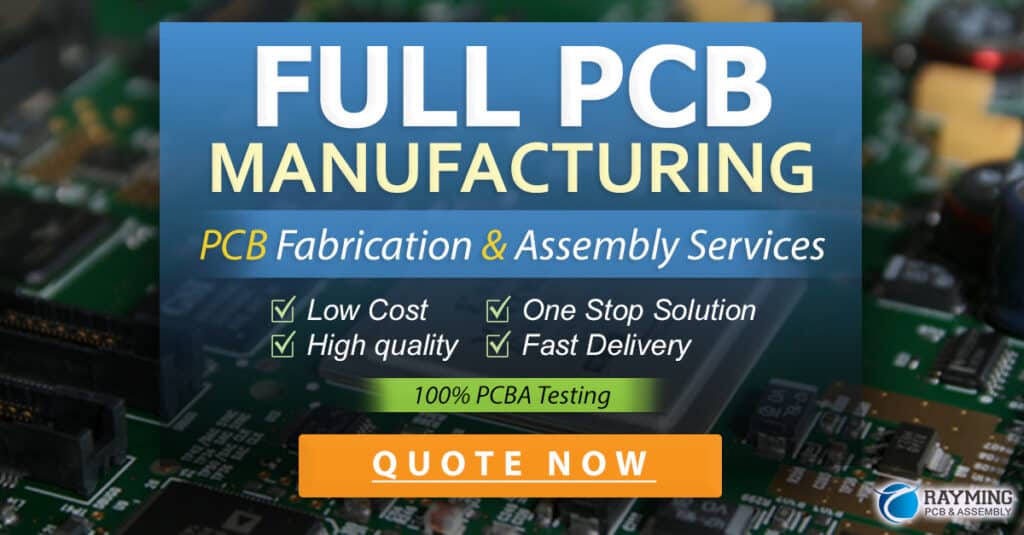
Conclusion
Spin-wave memory is a promising technology that has the potential to revolutionize the field of data storage and processing. With its unique combination of ultra-low power consumption, non-volatility, and high-speed operation, spin-wave memory could enable a new generation of energy-efficient and high-performance computing devices.
Recent breakthroughs in materials science, device engineering, and integration with CMOS technology have brought spin-wave memory closer to practical implementation. However, there are still several challenges that need to be addressed, including scalability, energy efficiency, speed and bandwidth, and reliability and endurance.
As research in this field continues to advance, we can expect to see further developments in spin-wave memory technology, bringing us closer to a future where this innovative approach becomes a mainstream solution for data storage and processing.
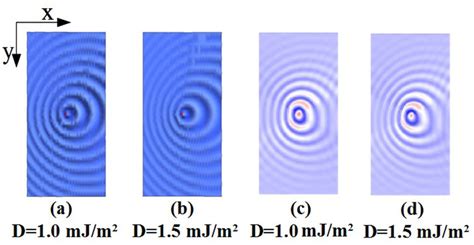
Frequently Asked Questions
-
What is spin-wave memory?
Spin-wave memory is a type of non-volatile memory that uses the collective oscillation of electron spins in magnetic materials to store and process information. -
How does spin-wave memory differ from traditional electronic memory?
Traditional electronic memory relies on the movement of electrons to store and process information, while spin-wave memory utilizes the collective oscillation of electron spins in magnetic materials. This difference enables spin-wave memory to achieve ultra-low power consumption and non-volatility. -
What are the advantages of spin-wave memory?
The main advantages of spin-wave memory are ultra-low power consumption, non-volatility, high-speed operation, and scalability. Spin waves can be excited with very low energy inputs, retain information even when the power is turned off, propagate at high speeds, and be confined to nanoscale dimensions. -
What are some of the challenges in developing spin-wave memory?
Some of the challenges in developing spin-wave memory include finding suitable materials that can efficiently generate, propagate, and detect spin waves, developing compact and integrated device architectures, improving energy efficiency, increasing speed and bandwidth, and ensuring reliability and endurance. -
What are the potential applications of spin-wave memory?
Spin-wave memory has the potential to be used in a wide range of applications, including ultra-low power IoT devices, high-performance computing, artificial intelligence, and neuromorphic computing. Its unique combination of low power consumption, non-volatility, and high-speed operation makes it an attractive solution for future data storage and processing needs.
No responses yet