Introduction to S-Parameters and Power Integrity
S-parameters, or scattering parameters, are a powerful tool for characterizing high-frequency electrical networks, including power distribution networks (PDNs) in electronic systems. In the context of power integrity (PI), S-parameters can provide valuable insights into the behavior of PDNs, helping designers to optimize their designs for better signal quality and power delivery.
However, measuring S-parameters accurately can be challenging, especially at high frequencies where various sources of error can affect the results. Understanding these errors and how to mitigate them is crucial for obtaining reliable S-parameter data and making informed design decisions.
In this article, we will explore the basics of S-parameters, their application in power integrity, and the common sources of error in S-parameter measurements. We will also discuss techniques for minimizing these errors and improving the accuracy of S-parameter data.
What are S-Parameters?
S-parameters are a mathematical representation of the scattering of electromagnetic waves in an electrical network. They describe the relationship between the incident and reflected waves at each port of the network, providing a complete characterization of its linear behavior.
For a two-port network, the S-parameters are defined as follows:
Parameter | Description |
---|---|
S11 | Input reflection coefficient |
S12 | Reverse transmission coefficient |
S21 | Forward transmission coefficient |
S22 | Output reflection coefficient |
These parameters can be measured using a vector network analyzer (VNA) and are typically expressed as complex numbers, with magnitude and phase information. S-parameters are frequency-dependent, meaning that their values change with the frequency of the incident signal.
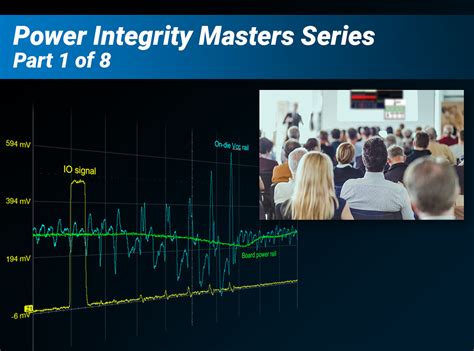
Why are S-Parameters Important for Power Integrity?
In power integrity analysis, S-parameters play a crucial role in characterizing the behavior of power distribution networks. PDNs are responsible for delivering clean and stable power to the active components in an electronic system, such as integrated circuits (ICs) and field-programmable gate arrays (FPGAs).
However, PDNs are not ideal and can exhibit complex impedance characteristics that vary with frequency. This can lead to voltage fluctuations, noise, and other power integrity issues that can affect the performance and reliability of the system.
By measuring the S-parameters of a PDN, designers can gain valuable insights into its impedance profile, resonances, and other key characteristics. This information can be used to optimize the PDN design, minimize impedance discontinuities, and ensure that the system meets its power integrity requirements.
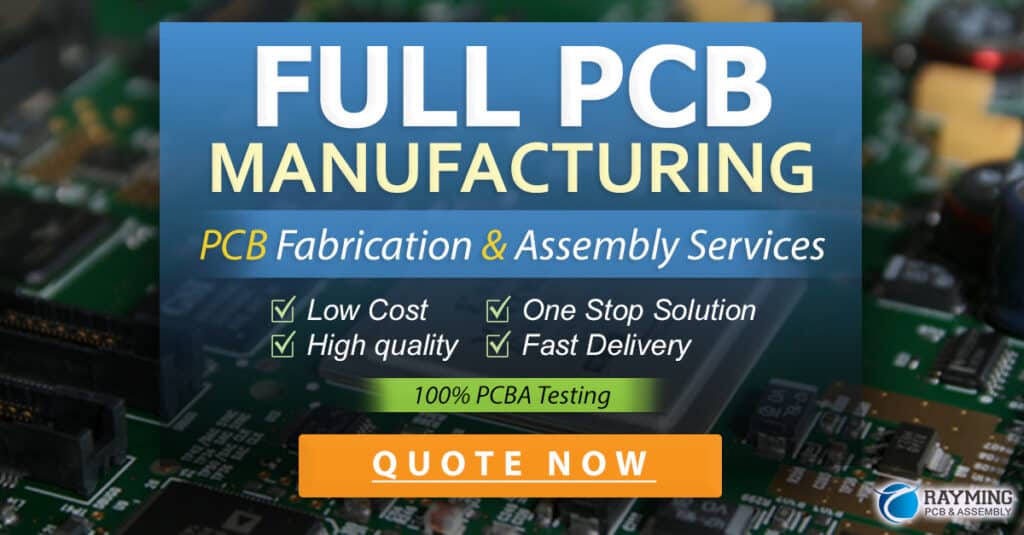
Sources of Error in S-Parameter Measurements
Accurate S-parameter measurements are essential for effective power integrity analysis and design optimization. However, various sources of error can affect the accuracy of these measurements, particularly at high frequencies. Some of the most common sources of error include:
1. Calibration Errors
Calibration is a critical step in S-parameter measurements, as it removes the effects of the measurement setup and cables from the measured data. However, poor calibration or drift in the calibration standards can introduce errors into the measurements.
To minimize calibration errors, it is important to use high-quality calibration standards and to perform regular calibration checks and updates. Techniques such as electronic calibration (eCal) can also help to improve calibration accuracy and repeatability.
2. Cable and Connector Issues
Cables and connectors can introduce significant errors into S-parameter measurements, especially at high frequencies. Factors such as cable loss, impedance mismatch, and connector discontinuities can distort the measured data and lead to inaccurate results.
To mitigate these errors, it is important to use high-quality cables and connectors that are rated for the desired frequency range. Proper cable management, such as minimizing cable bends and avoiding excessive cable length, can also help to reduce measurement errors.
3. Probe Placement and Contact Issues
When measuring S-parameters using probes, the placement and contact quality of the probes can have a significant impact on the accuracy of the measurements. Poor probe placement or inconsistent contact can introduce errors and distort the measured data.
To minimize these errors, it is important to use appropriate probing techniques and to ensure consistent probe placement and contact. High-quality probes with reliable contact mechanisms can also help to improve measurement accuracy.
4. Fixture and PCB Effects
The test fixture and printed circuit board (PCB) can also introduce errors into S-parameter measurements. Factors such as impedance discontinuities, parasitic inductance and capacitance, and coupling between traces can distort the measured data.
To mitigate these errors, it is important to use well-designed test fixtures and PCBs that minimize the impact of these factors. Techniques such as de-embedding can also be used to remove the effects of the fixture and PCB from the measured data.
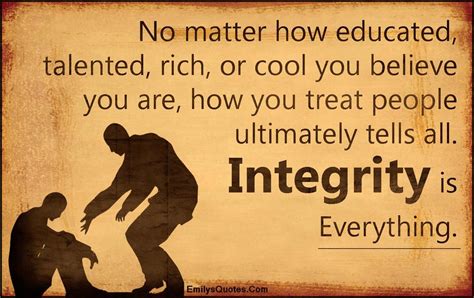
Techniques for Improving S-Parameter Measurement Accuracy
While various sources of error can affect the accuracy of S-parameter measurements, there are several techniques that can be used to minimize these errors and improve the quality of the measured data. Some of the most effective techniques include:
1. Proper Calibration and Calibration Checks
As mentioned earlier, proper calibration is essential for accurate S-parameter measurements. Performing regular calibration checks and updates can help to ensure that the calibration remains valid and minimize drift over time.
Using high-quality calibration standards and techniques such as electronic calibration (eCal) can also help to improve calibration accuracy and repeatability.
2. De-embedding Techniques
De-embedding is a technique that removes the effects of the test fixture and PCB from the measured S-parameter data. By characterizing the fixture and PCB separately and subtracting their effects from the measured data, de-embedding can provide a more accurate representation of the device under test (DUT).
There are various de-embedding techniques available, such as time-domain reflectometry (TDR) based de-embedding and 2x-Thru de-embedding. Choosing the appropriate de-embedding technique for a given measurement scenario can help to improve the accuracy of the results.
3. Averaging and Smoothing
Averaging and smoothing are techniques that can be used to reduce the impact of random noise and measurement variations on S-parameter data. By averaging multiple measurements or applying smoothing algorithms to the data, designers can obtain a more stable and consistent representation of the device’s behavior.
However, it is important to use these techniques judiciously, as excessive averaging or smoothing can also remove important features and details from the data.
4. Advanced Calibration Techniques
In addition to traditional calibration methods, there are several advanced calibration techniques that can be used to further improve the accuracy of S-parameter measurements. These include:
- Adapter removal calibration: This technique removes the effects of adapters and connectors from the measured data, providing a more accurate representation of the DUT.
- Vector receiver calibration (VRC): VRC is a technique that calibrates the magnitude and phase response of the VNA’s receivers, improving the accuracy of the measured data.
- Nonlinear calibration: This technique accounts for the nonlinear behavior of the VNA and the measurement setup, providing more accurate results at high power levels.
By using these advanced calibration techniques in combination with proper measurement practices, designers can obtain highly accurate S-parameter data for power integrity analysis and optimization.
FAQ
1. What is the difference between S-parameters and Z-parameters?
S-parameters and Z-parameters are both used to characterize the behavior of electrical networks, but they represent different aspects of the network’s response. S-parameters describe the scattering of electromagnetic waves in the network, while Z-parameters represent the impedance of the network.
S-parameters are typically used for high-frequency measurements, where the wavelength of the signal is comparable to or smaller than the size of the network. Z-parameters are more commonly used for low-frequency measurements, where the network can be treated as a lumped-element model.
2. Can S-parameters be used for time-domain analysis?
Yes, S-parameters can be used for time-domain analysis through a process called inverse Fourier transform (IFT). By applying an IFT to the frequency-domain S-parameter data, designers can obtain the time-domain response of the network, such as the impulse response or step response.
Time-domain analysis can provide valuable insights into the transient behavior of the network, such as the rise time, overshoot, and settling time of the signal. This information can be used to optimize the design for better signal integrity and power integrity performance.
3. How do I choose the appropriate frequency range for S-parameter measurements?
The appropriate frequency range for S-parameter measurements depends on the specific application and the characteristics of the device under test (DUT). In general, the frequency range should be wide enough to capture the relevant behavior of the DUT, but not so wide that it introduces unnecessary measurement errors or complexity.
For power integrity applications, the frequency range of interest typically extends from DC to several gigahertz, depending on the speed and bandwidth of the system. It is important to consider the frequency content of the power supply noise, as well as the resonances and impedance characteristics of the power distribution network, when selecting the appropriate frequency range.
4. What is the impact of measurement bandwidth on S-parameter accuracy?
Measurement bandwidth, also known as intermediate frequency (IF) bandwidth, is a key parameter in S-parameter measurements that affects the accuracy and noise level of the results. A wider measurement bandwidth allows for faster measurements but also increases the noise floor, while a narrower bandwidth provides lower noise but slower measurements.
The choice of measurement bandwidth depends on the specific requirements of the application, such as the desired measurement speed, dynamic range, and sensitivity. In general, it is recommended to use the narrowest bandwidth that provides acceptable measurement speed and noise performance for a given application.
5. How can I determine the quality of my S-parameter measurements?
There are several metrics that can be used to assess the quality of S-parameter measurements, including:
- Magnitude and phase accuracy: This can be evaluated by comparing the measured data to known reference standards or by performing cross-comparisons between different measurement setups.
- Repeatability: Repeating the same measurement multiple times and comparing the results can provide an indication of the measurement stability and reproducibility.
- Dynamic range: The dynamic range of the measurement setup, defined as the ratio between the maximum and minimum measurable signals, can affect the accuracy and sensitivity of the results.
- Noise floor: The noise floor of the measurement setup determines the minimum signal level that can be accurately measured and can impact the dynamic range and sensitivity of the results.
By monitoring these metrics and performing regular quality checks, designers can ensure that their S-parameter measurements are reliable and accurate for power integrity analysis and optimization.
Conclusion
S-parameter measurements are a powerful tool for characterizing the behavior of power distribution networks and optimizing their design for better power integrity performance. However, accurate S-parameter measurements can be challenging, especially at high frequencies where various sources of error can affect the results.
By understanding these sources of error and employing techniques such as proper calibration, de-embedding, averaging, and advanced calibration methods, designers can minimize the impact of these errors and obtain reliable S-parameter data for their power integrity analysis and optimization.
As power integrity becomes increasingly critical in modern electronic systems, the ability to accurately measure and analyze S-parameters will be essential for ensuring the performance, reliability, and efficiency of these systems. By staying up-to-date with the latest measurement techniques and best practices, designers can effectively address the power integrity challenges of today and tomorrow.
No responses yet