Introduction to Phased Array Antennas
Phased array antennas are a key enabling technology for 5G wireless networks. Unlike traditional antennas that radiate signals in a fixed direction, phased arrays can dynamically steer beams of radio waves to track mobile users and optimize signal quality. This beam-steering capability allows 5G base stations to provide faster data speeds, lower latency, and support more simultaneous users compared to 4G networks.
A phased array consists of multiple antenna elements arranged in a grid. By adjusting the relative phase and amplitude of the signal feeding each element, the radiation pattern of the overall array can be shaped and directed as desired. This is accomplished through an electronic beamforming network that controls the phase shifters and attenuators connected to each antenna.
Advantages of Phased Arrays for 5G
Phased array antennas offer several key benefits for 5G applications:
- Increased antenna gain and directivity for longer range
- Dynamic beam steering to track users and mitigate interference
- Ability to form multiple beams to serve more users
- Compact size compared to mechanically steered dish antennas
- Lower power consumption and longer battery life in mobile devices
5G Frequency Bands and Phased Array Design Considerations
5G networks will utilize several new frequency bands to provide enhanced mobile broadband (eMBB), ultra-reliable low-latency communication (URLLC), and massive machine-type communication (mMTC) services. The main 5G bands can be grouped into low-band (sub-1 GHz), mid-band (1-6 GHz), and high-band (above 24 GHz) frequencies.
5G Band | Frequency Range | Primary Applications |
---|---|---|
Low-band | 600-700 MHz | Wide area coverage, IoT |
Mid-band | 3.3-4.2 GHz, 4.4-5.0 GHz | eMBB, URLLC, fixed wireless |
High-band (mmWave) | 24-29.5 GHz, 37-43.5 GHz | eMBB, indoor hotspots |
The choice of frequency band impacts several key design parameters for 5G phased array antennas:
Array Size and Element Spacing
At lower 5G frequencies (e.g. 3-4 GHz), larger arrays with more elements are needed to achieve sufficient gain and directivity. The spacing between elements is typically set to half the wavelength (λ/2) to avoid grating lobes. For example, a 64-element array operating at 4 GHz (λ = 7.5 cm) would require an aperture size of about 30 cm x 30 cm.
At mmWave frequencies, the wavelength is much smaller, allowing for compact, high-gain arrays. A 256-element array at 28 GHz (λ = 10.7 mm) could fit within a 10 cm x 10 cm aperture. However, the smaller feature sizes require advanced manufacturing techniques and high-performance phase shifters.
Beamwidth and Scanning Range
The beamwidth of a phased array scales inversely with the electrical size of the aperture (D/λ). Larger arrays produce narrower beams for higher directivity. The maximum scanning range depends on the element pattern and spacing. Wide-angle scanning requires elements with broad radiation patterns and spacing less than λ/2 to avoid grating lobes.
Typical phased array specifications for different 5G bands are:
5G Band | Array Size | Beamwidth | Scan Range |
---|---|---|---|
Mid-band (3-4 GHz) | 64-256 elements | 5°-15° | ±60° |
mmWave (24-30 GHz) | 256-1024 elements | 2°-5° | ±45° |
Phase Shifters and Time-Delay Units
Phase shifters are a critical component in phased array antennas, controlling the relative phase of each element to steer the beam. At lower 5G frequencies, analog phase shifters based on RF MEMS, PIN diodes, or vector modulators are commonly used. These provide continuous phase control but have limited bandwidth and require careful calibration.
For wideband mmWave arrays, true-time-delay units (TTDs) are preferred over phase shifters. TTDs introduce a frequency-independent time delay to each element, allowing the beam to be steered without squinting or distortion over a wide bandwidth. However, TTDs are more complex and expensive to implement than phase shifters.
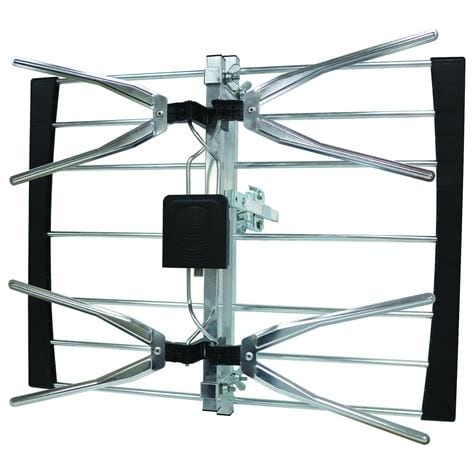
Antenna Elements for 5G Phased Arrays
The type of antenna element used in a 5G phased array depends on the frequency band, polarization, and scan requirements. Common element types include:
Patch Antennas
Microstrip patch antennas are widely used in sub-6 GHz arrays due to their low profile, low cost, and ease of fabrication. They consist of a metal patch printed on a dielectric substrate over a ground plane. Patches can be linearly or circularly polarized and have moderate bandwidth (5-10%).
Dipole Antennas
Dipoles are another popular choice for sub-6 GHz arrays. They offer wide bandwidth, low cross-polarization, and omnidirectional patterns suitable for wide-angle scanning. Printed dipoles can be integrated with baluns and feeding networks on a PCB.
Vivaldi Antennas
For wideband mmWave applications, tapered slot antennas like Vivaldi antennas are often used. They provide wide bandwidth (>30%), high gain, and endfire radiation patterns. Vivaldi antennas can be etched on a substrate or machined from a metal block.
Waveguide Antenna Arrays
At higher mmWave frequencies (above 40 GHz), waveguide-based antenna arrays offer low loss and high power handling. They consist of an array of slot or horn antennas fed by a corporate waveguide network. Waveguide arrays have high efficiency but are bulky and expensive to manufacture.
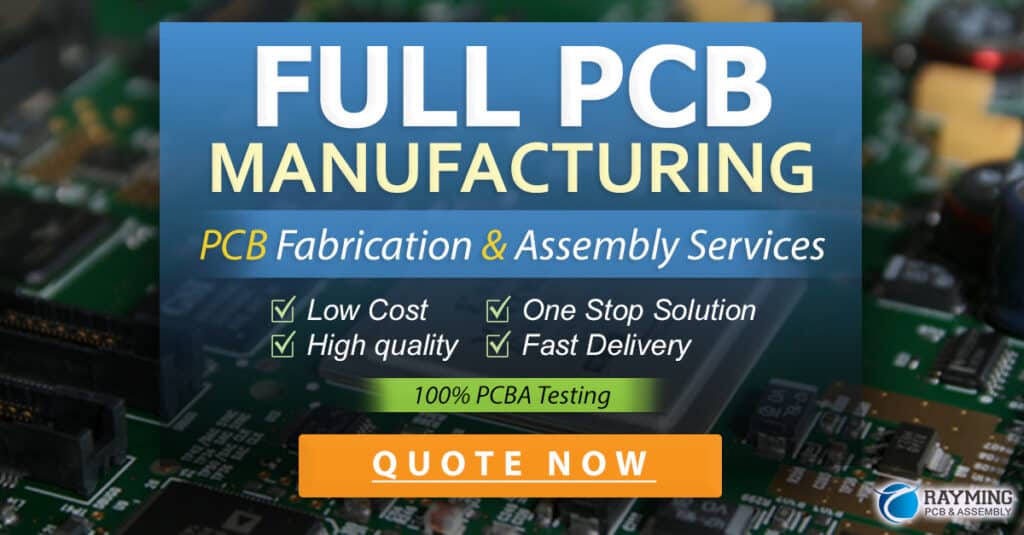
Feeding Networks for 5G Phased Arrays
The feeding network distributes the RF signal from the transceiver to the individual antenna elements with the desired phase and amplitude weighting. The choice of feeding topology impacts the array’s bandwidth, loss, and beam-steering performance.
Corporate Feed Networks
In a corporate feed, the RF signal is divided into equal-amplitude, equal-phase branches using power dividers or hybrid couplers. Each branch feeds a subarray of elements through a phase shifter and attenuator. Corporate feeds provide good amplitude and phase control but have high loss and limited bandwidth.
Series Feed Networks
Series feeds, also known as traveling wave feeds, distribute the signal along a transmission line with taps or couplers to feed each element. They have lower loss and wider bandwidth than corporate feeds but provide limited phase control and are more prone to beam squint.
Optical Beamforming Networks
For wideband mmWave arrays, RF feeding networks become lossy and complex. An alternative approach is to use an optical beamforming network, where the phase and amplitude control is performed in the optical domain. In this scheme, the RF signal modulates an optical carrier, which is split and delayed using optical phase shifters or TTDs before being converted back to RF at each element. Optical beamforming offers ultra-wide bandwidth and low loss but requires complex photonic integrated circuits.
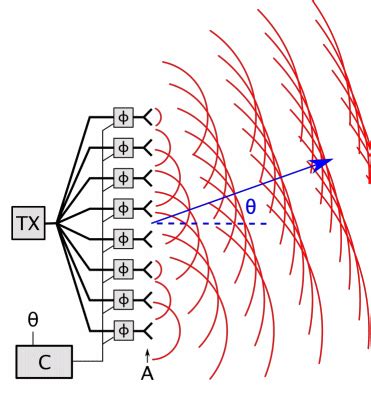
Performance Metrics for 5G Phased Array Antennas
Several key performance metrics characterize the operation of 5G phased array antennas:
Radiation Pattern and Directivity
The radiation pattern describes the angular distribution of the radiated power. It is characterized by the main beam width, sidelobe levels, and null depths. Phased arrays can achieve high directivity (>30 dBi) by coherently combining the fields from multiple elements.
Gain and Efficiency
The realized gain of a phased array accounts for the directivity, element efficiency, and feeding network losses. Typical gains for 5G arrays range from 20-40 dBi. The aperture efficiency measures how effectively the array captures incoming radiation and is affected by element spacing, amplitude tapering, and scan loss.
Beam-Steering Speed and Accuracy
The beam-steering speed determines how quickly the array can redirect its beam to track moving users or switch between multiple beams. It is limited by the response time of the phase shifters and control circuitry. The steering accuracy depends on the phase and amplitude resolution of the beamforming network and the calibration of the array.
Bandwidth and Frequency Agility
The operating bandwidth of a phased array dictates the range of frequencies over which it can maintain a stable beam pattern and impedance match. Wideband arrays for 5G require elements with low Q-factor, broadband feeding networks, and TTDs for squint-free steering. Frequency-agile arrays can rapidly tune their operating frequency to avoid interference or exploit spectrum holes.
Fabrication and Integration Technologies for 5G Phased Arrays
The choice of fabrication technology for 5G phased arrays depends on the frequency band, array size, and integration level required. Commonly used technologies include:
Printed Circuit Board (PCB)
PCB-based arrays are widely used for sub-6 GHz applications. They consist of patch or dipole elements, phase shifters, and feeding networks printed on a laminate substrate. PCB arrays are low-cost and easy to manufacture but have limited performance at higher frequencies.
Low Temperature Co-fired Ceramic (LTCC)
LTCC technology allows for 3D integration of antenna elements, phase shifters, and MMIC components in a multilayer ceramic package. It offers higher density and better performance than PCB at mmWave frequencies but is more expensive.
Monolithic Microwave Integrated Circuits (MMIC)
MMICs integrate the phase shifters, attenuators, amplifiers, and switches on a single semiconductor chip, typically GaAs or GaN. They enable compact, low-power phased arrays for mobile devices but are costly to develop and manufacture.
Advanced Packaging and Interconnects
At mmWave frequencies, the interconnects between the antenna elements and RFIC become a critical bottleneck. Advanced packaging techniques like antenna-in-package (AiP) and wafer-level packaging (WLP) reduce interconnect loss and enable higher integration. Novel interconnect technologies like silicon vias, air bridges, and flip-chip bonding are also being developed for mmWave arrays.
Future Trends and Challenges in 5G Phased Array Design
As 5G networks continue to evolve, phased array technology will face new challenges and opportunities:
Digital Beamforming and Massive MIMO
Digital beamforming relies on dedicated RF chains and data converters for each antenna element, allowing for more flexible and dynamic beamforming than analog phased arrays. Combined with massive MIMO techniques, digital beamforming can enable multi-user MIMO, spatial multiplexing, and more sophisticated interference mitigation schemes. However, the power consumption and cost of digital beamforming remains a challenge, especially at mmWave frequencies.
Hybrid Beamforming Architectures
Hybrid beamforming architectures split the beamforming function between the analog and digital domains. They use a smaller number of RF chains and a phase-shifting network to partially form beams in the analog domain, followed by digital precoding to fine-tune the beams and manage interference. Hybrid beamforming provides a balance between the flexibility of digital beamforming and the efficiency of analog phased arrays.
Software-Defined Arrays and Artificial Intelligence
Future phased arrays will incorporate more software-defined and cognitive capabilities. Software-defined arrays use programmable hardware like FPGAs or ASICs to adapt the beamforming weights and array configuration in real-time. Machine learning algorithms can be used to optimize the array performance based on channel conditions, user demands, and network state. AI-driven phased arrays could autonomously learn to form beams, avoid interference, and allocate resources in dynamic environments.
Integrated Sensing and Communication
5G phased arrays can be used for both communication and sensing applications like radar, imaging, and localization. By sharing hardware and spectrum resources between these functions, integrated sensing and communication (ISAC) systems can provide enhanced situational awareness and enable new applications like vehicle-to-everything (V2X) communication, gesture recognition, and security monitoring.
Terahertz and Optical Beamforming
As data rates continue to increase, future wireless networks may move to even higher frequencies in the terahertz (100-1000 GHz) or optical (free-space laser communication) bands. Phased arrays for these frequencies will require novel materials, devices, and beamforming architectures. Graphene-based plasmonic antennas, metamaterial lenses, and optical phased arrays are some of the emerging technologies being explored for ultra-high frequency beamforming.
Frequently Asked Questions (FAQ)
What is a phased array antenna and how does it work?
A: A phased array antenna consists of multiple antenna elements that are fed with signals of different phases, allowing the beam pattern to be electronically steered without physically moving the antenna. By adjusting the relative phase shift between elements, the radiation from each element interferes constructively in the desired direction and destructively elsewhere, focusing the energy into a directional beam. This enables the antenna to dynamically track users and steer nulls towards interferers.
What are the key advantages of phased arrays for 5G?
A: Phased arrays offer several benefits for 5G networks, including:
– High gain and directivity for longer range and higher data rates
– Dynamic beam steering to track mobile users and adapt to changing environments
– Multi-beam capability to serve multiple users simultaneously
– Compact size and low visual impact compared to mechanically steered antennas
– Potential for integration with other RF components like transceivers and amplifiers
What is the impact of 5G frequency bands on phased array design?
A: The choice of 5G frequency band affects several key parameters of phased array design, such as:
– Array size and number of elements: higher frequencies allow for smaller, more compact arrays
– Element spacing and scan range: wide-angle scanning requires closer element spacing (<0.5 wavelength)
– Phase shifter or time-delay unit technology: mmWave arrays may require true-time delay for wideband operation
– RF losses and power handling: mmWave frequencies have higher loss and require more advanced materials and packaging technologies
What are some of the challenges in developing phased arrays for 5G?
A: Some of the key challenges include:
– Designing wideband, low-loss antenna elements and feeding networks for mmWave frequencies
– Developing low-cost, high-performance phase shifters and RF front-ends
– Integrating the antenna array with the transceiver and signal processing circuitry
– Managing the power consumption and heat dissipation of large arrays
– Calibrating and testing the array performance over wide bandwidths and scan angles
– Adapting the beamforming algorithms to changing channel conditions and user demands
What are some of the emerging trends in phased array technology for 5G and beyond?
A: Some of the key trends shaping the future of phased arrays for wireless communications include:
– Digital beamforming and massive MIMO architectures for higher capacity and flexibility
– Hybrid analog-digital beamforming for a balance of performance and complexity
– Software-defined and AI-driven arrays that can adapt and learn from their environment
– Integrated sensing and communication (ISAC) systems that share hardware and spectrum between radar and communications
– Migration to higher frequencies (terahertz and optical) for ultra-high data rates and new applications
Phased array antennas will continue to play a critical role in the evolution of 5G and future wireless networks, enabling higher performance, flexibility, and adaptability to meet the growing demands for connectivity and sensing in the digital world.
No responses yet