What is Piezoelectricity?
Piezoelectricity is a property exhibited by certain materials that generate an electric charge when subjected to mechanical stress or strain. Conversely, these materials also experience mechanical deformation when an electric field is applied to them. This reciprocal relationship between mechanical and electrical energy forms the basis of the piezoelectric effect.
The term “piezoelectricity” is derived from the Greek word “piezein,” which means to press or squeeze, and “elektron,” referring to electricity. The piezoelectric effect was first discovered by Pierre and Jacques Curie in 1880 during their study of crystalline materials.
Piezoelectric Materials
Not all materials exhibit piezoelectric properties. Piezoelectric materials are typically crystalline or ceramic in nature and have a non-centrosymmetric crystal structure. This means that the positive and negative charges within the material are not symmetrically distributed, leading to the formation of electric dipoles.
Some common piezoelectric materials include:
- Quartz (SiO2)
- Lead Zirconate Titanate (PZT)
- Barium Titanate (BaTiO3)
- Polyvinylidene Fluoride (PVDF)
- Zinc Oxide (ZnO)
- Aluminum Nitride (AlN)
Each piezoelectric material has its own unique properties and characteristics, making them suitable for different applications.
Piezoelectric Effect: Direct and Converse
The piezoelectric effect can be categorized into two types: the direct piezoelectric effect and the converse piezoelectric effect.
Direct Piezoelectric Effect
The direct piezoelectric effect refers to the generation of an electric charge when a piezoelectric material is subjected to mechanical stress or strain. When the material is compressed or stretched, the electric dipoles within the crystal structure align, causing a net polarization and the accumulation of charges on the material’s surfaces. This generated electric charge is proportional to the applied mechanical stress.
Converse Piezoelectric Effect
The converse piezoelectric effect describes the mechanical deformation of a piezoelectric material when an electric field is applied to it. When an external electric field is applied, the electric dipoles within the material align with the field, causing the material to expand or contract. The extent of the mechanical deformation depends on the strength and polarity of the applied electric field.
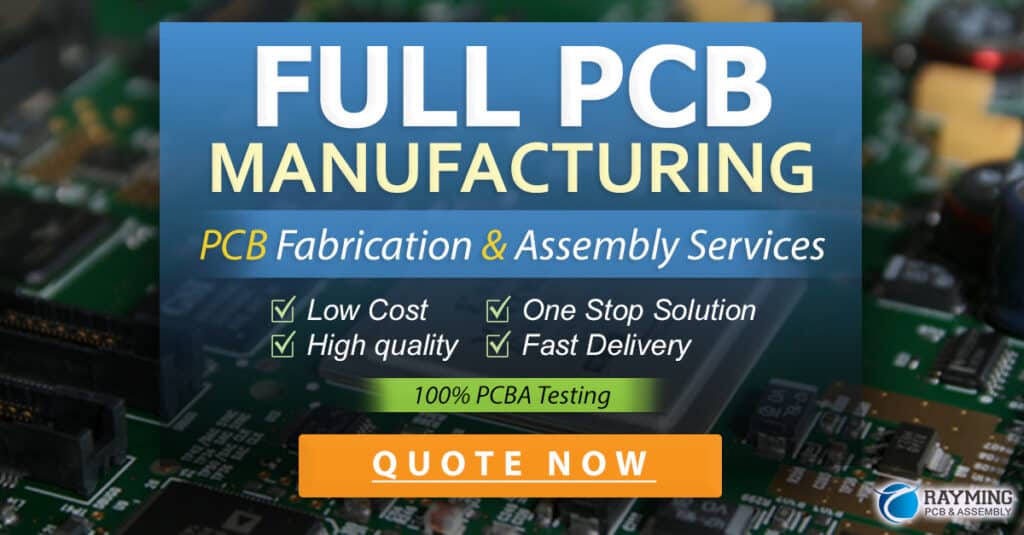
Piezoelectric Coefficients
To quantify the piezoelectric properties of a material, several piezoelectric coefficients are used. These coefficients relate the mechanical and electrical quantities in a piezoelectric material.
The most commonly used piezoelectric coefficients are:
-
d-coefficient (Piezoelectric Charge Constant): It represents the ratio of the generated electric charge to the applied mechanical stress or the mechanical strain produced per unit of applied electric field. The d-coefficient is expressed in units of C/N (Coulomb per Newton) or m/V (meter per Volt).
-
g-coefficient (Piezoelectric Voltage Constant): It represents the ratio of the open-circuit electric field generated per unit of applied mechanical stress or the mechanical strain produced per unit of applied electric displacement. The g-coefficient is expressed in units of Vm/N (Volt-meter per Newton) or m2/C (square meter per Coulomb).
-
k-coefficient (Electromechanical Coupling Factor): It is a dimensionless quantity that indicates the efficiency of a piezoelectric material in converting mechanical energy to electrical energy or vice versa. The k-coefficient ranges from 0 to 1, with higher values indicating better energy conversion efficiency.
Here’s a table summarizing the piezoelectric coefficients:
Coefficient | Symbol | Units | Description |
---|---|---|---|
Piezoelectric Charge Constant | d | C/N or m/V | Generated electric charge per unit of applied mechanical stress or mechanical strain per unit of applied electric field |
Piezoelectric Voltage Constant | g | Vm/N or m2/C | Generated open-circuit electric field per unit of applied mechanical stress or mechanical strain per unit of applied electric displacement |
Electromechanical Coupling Factor | k | Dimensionless | Efficiency of energy conversion between mechanical and electrical domains |
Piezoelectric Applications
The unique properties of piezoelectric materials have led to their widespread use in various applications. Some of the key applications of piezoelectricity include:
Sensors
Piezoelectric materials are extensively used in sensing applications due to their ability to generate an electric charge in response to mechanical stimuli. They are employed in various types of sensors, such as:
- Pressure sensors
- Acceleration sensors
- Strain gauges
- Vibration Sensors
- Acoustic sensors
These sensors find applications in industries such as automotive, aerospace, industrial automation, and medical devices.
Actuators
Piezoelectric actuators leverage the converse piezoelectric effect to convert electrical energy into precise mechanical motion. They offer high-precision positioning, fast response times, and high force generation capabilities. Piezoelectric actuators are used in:
- Precision positioning systems
- Micro- and nanopositioning stages
- Fuel injectors
- Inkjet printer heads
- Ultrasonic motors
Transducers
Piezoelectric transducers convert electrical energy into mechanical energy (sound or ultrasound) and vice versa. They are commonly used in:
- Ultrasonic cleaning devices
- Sonar systems
- Medical ultrasound imaging
- Non-destructive testing (NDT) equipment
- Ultrasonic welding machines
Energy Harvesting
Piezoelectric materials can convert ambient mechanical energy, such as vibrations or pressure fluctuations, into electrical energy. This makes them attractive for energy harvesting applications, where the generated electrical energy can power small electronic devices or sensors. Piezoelectric energy harvesting finds applications in:
- Wearable electronics
- Wireless sensor networks
- Structural health monitoring
- Tire pressure monitoring systems
- Self-powered electronic devices
Advantages and Limitations of Piezoelectric Materials
Piezoelectric materials offer several advantages, including:
- High sensitivity and responsiveness
- Wide frequency range of operation
- Compact size and lightweight
- Low power consumption
- Ability to generate high voltages
- Durability and reliability
However, piezoelectric materials also have some limitations:
- Limited strain and displacement range
- Temperature sensitivity
- Depolarization at high temperatures or under high mechanical stress
- Brittleness of some piezoelectric ceramics
- High cost of certain piezoelectric materials
Frequently Asked Questions (FAQ)
-
Q: Can piezoelectric materials generate electricity continuously?
A: No, piezoelectric materials generate electricity only when subjected to changing mechanical stress or strain. They do not generate a continuous output unless there is a continuous variation in the applied mechanical input. -
Q: Are piezoelectric materials used in everyday life?
A: Yes, piezoelectric materials are used in many everyday applications, such as ignitors in gas lighters, quartz watches, microphones, and ultrasonic toothbrushes. -
Q: How long do piezoelectric materials last?
A: The lifespan of piezoelectric materials depends on various factors, such as the material composition, operating conditions, and the magnitude of applied mechanical and electrical stresses. With proper usage and maintenance, piezoelectric devices can last for many years. -
Q: Can piezoelectric materials be used for large-scale energy generation?
A: While piezoelectric materials are effective for small-scale energy harvesting, their power output is relatively low for large-scale energy generation. They are more suitable for powering small electronic devices or sensors rather than generating significant amounts of electricity. -
Q: Are piezoelectric materials environmentally friendly?
A: Most piezoelectric materials are non-toxic and do not contain hazardous substances. However, some piezoelectric ceramics, such as lead zirconate titanate (PZT), contain lead, which can be harmful if not disposed of properly. Researchers are actively developing lead-free piezoelectric materials to address environmental concerns.
Conclusion
Piezoelectric materials have revolutionized the way we convert mechanical energy into electrical energy and vice versa. Their unique properties have enabled the development of a wide range of applications, from sensors and actuators to energy harvesting devices. As research continues to advance, we can expect to see further improvements in piezoelectric materials and their integration into innovative technologies.
Understanding the fundamentals of piezoelectricity, its working principles, and the various piezoelectric coefficients is crucial for engineers, researchers, and enthusiasts working in this field. By harnessing the power of piezoelectric materials, we can create smarter, more efficient, and sustainable solutions for a wide range of industries.
This ultimate guide has provided a comprehensive overview of piezoelectricity, covering its basic concepts, material properties, applications, advantages, and limitations. With this knowledge, you are well-equipped to explore the exciting world of piezoelectric materials and contribute to the development of cutting-edge technologies that leverage this fascinating phenomenon.
No responses yet